Semi-supervised aerobic interval training improves cardiorespiratory fitness in sedentary adults with cardiometabolic risk factors
*Corresponding author: Leonardo Machado leonardoed.fisica@hotmail.com
Cite this article
Ensenyat, A., Machado, L., Matas, S. & Blanco, A. (2023). Semi-supervised aerobic interval training improves cardiorespiratory fitness in sedentary adults with cardiometabolic risk factors.Apunts Educación Física y Deportes, 154, 1-15. https://doi.org/10.5672/apunts.2014-0983.es.(2023/4).154.01
Abstract
The aim of this study was to evaluate the effects of a high-intensity semi-supervised exercise programme alongside lifestyle counselling on cardiorespiratory adaptation to exertion in sedentary adults with cardiometabolic risk factors. Forty-week three-arm randomized controlled clinical trial (16-week intervention and 24-week follow-up). Sedentary adults (23 men, 38 women) aged 34-to-52 years (M = 44.6, SD = 4.6), with at least one cardiometabolic risk factor were randomized into one of the following arms: (1) aerobic interval training plus lifestyle counselling (AIT); (2) low-to-moderate-intensity continuous training plus lifestyle counselling (TCT); or (3) lifestyle counselling alone (COU). Cardiorespiratory fitness, in terms of peak oxygen uptake (VO2peak) and oxygen uptake at the respiratory compensation point (VO2RCP), ventilatory efficiency index (VE-VCO2 slope), oxygen uptake efficiency slope (OUES), were assessed at baseline, after the intervention and at follow-up. All intervention programmes induced comparable significant changes in cardiorespiratory fitness, but changes in VO2peak differed according to whether participants could reach maximal criteria at baseline or not. Intervention was more effective for participants that were unable to reach maximal criteria at baseline than for those that reached maximal criteria. In participants that did not reach maximal criteria at baseline, VO2peak increased significantly. All intervention programmes induced comparable significant but non-persistent increases in VO2VT, no changes in VE-VCO2 slope and persistent improvements of OUES. Semi-supervised AIT intervention had positive effects on physiological adaptation to exertion and cardiorespiratory fitness but did not differ substantially from TCT or COU.
Introduction
Metabolic syndrome (MetS), a cluster of cardiometabolic risk factors, has a strong negative impact on cardiovascular health and premature death (Gami et al., 2007). Epidemiological evidence indicates that unhealthy lifestyles are closely linked to the increasing prevalence of MetS and other non-communicable diseases worldwide (WHO, 2018). Lifestyle modifications have shown positive results in preventing and managing cardiometabolic risk (Pattyn et al., 2013).
Physical activity is considered a keystone of a healthy lifestyle and the health benefits of moderate-intensity exercise are well established (Pedersen & Saltin, 2015). However, for some years now, there has been growing interest in high-intensity interval training (HIIT), particularly aerobic interval training (AIT), in real-healthcare settings (Pattyn et al., 2013). Current evidence has revealed the potential of AIT as a valuable strategy to reduce the prevalence of cardiometabolic risk factors (Tjønna et al., 2018).
The level of cardiorespiratory fitness (CRF) has also been identified as an independent and strong predictor of mortality (Lee et al., 2010; Myers et al., 2015). In a recent systematic review, Lee (2020) reports that regardless of obesity status, low CRF was associated with 3.59 times higher metabolic syndrome risk, while high CRF decreased it by 77%. According to Knaeps et al. (2018), a combination of decreasing sedentary behaviour (SB) and increasing moderate-to-vigorous physical activity (MVPA), resulting in positive change in CRF, is likely to be most beneficial for cardiac and metabolic health.
CRF is commonly assessed by means of peak oxygen uptake (VO2peak) measured during cardiopulmonary exercise testing (CPET). An increase in VO2peak of 3.5 mL/kg/min (1-MET: metabolic equivalent) results in an improvement in survival of between 10% and 25% in both genders (Myers et al., 2015). It has been reported that the reduction in mortality may be even higher (30% per 1-MET increase) in low-fit individuals (<5 MET of functional capacity) with cardiovascular disease that participate in cardiac rehabilitation programmes (Myers et al., 2015).
AIT has consistently shown better results than traditional continuous training (TCT) when it comes to improving VO2peak (Pattyn et al., 2014; Wisløff et al., 2007). Given that low VO2peak is associated with premature mortality and cardiovascular risk (Lee, 2020; Myers et al., 2015), the potential ability of AIT to improve this parameter is, from the health perspective, a promising finding.
Besides VO2peak, CPET allows to assess several indices that are relatively independent of maximal effort, including the ventilatory efficiency index (ventilation vs. carbon dioxide output slope (VE-VCO2 slope)) and the oxygen uptake efficiency slope (OUES) (Baba et al., 1996). These indices are integrated measurements of physiological adaptation to exercise. They have prognostic value, are sensitive to change and relevant to evaluate the progression of the exercise capacity in patients with cardiac disease or mitochondrial myopathy (Akkerman et al., 2010). To our knowledge, there are few studies that evaluate the effects of HIIT interventions on these indices in individuals with metabolic syndrome (Guio de Prada et al., 2019a).
As a final point, although the efficacy of AIT to induce physiological improvements in a well-controlled research environment is no longer in doubt, questions remain regarding the extent to which its benefits are transferable to a less-controlled clinical environment (effectiveness). The evidence regarding the effectiveness of interventions promoting physical activity is inconsistent, because frequently exercise programmes have large dropout rates after a few weeks or months. Moreover, most studies have focussed on the short-term efficacy, but have not reported the persistence of effects. However, to achieve long-lasting and successful outcomes, these programmes need to include actions to help individuals enhance their self-efficacy, improving literacy and providing self-control tools (WHO, 2018). In this regard, our study analyses the short and medium-term effects and includes a lifestyle counselling intervention that incorporates strategies for promoting regular physical activity, minimizing sedentary behaviour, improving dietary habits, and enhancing participants’ self-efficacy.
The primary aim of this study was to evaluate the effectiveness of a semi-supervised aerobic interval training programme alongside lifestyle counselling (AIT) on cardiorespiratory fitness in terms of VO2peak, oxygen uptake at the respiratory compensation point (VO2RCP), VE-VCO2 slope and OUES in sedentary adults with cardiometabolic risk factors. This intervention was compared to low-to-moderate-intensity continuous training plus lifestyle counselling (TCT) and to lifestyle counselling (COU) alone. It was hypothesized that AIT would exhibit better results in terms of improving CRF than TCT or COU.
Materials and Methods
Design
A three-arm randomized controlled clinical trial (NCT02832453) was implemented in a primary care setting over a period of 16 weeks, with a 24-week follow-up. It included two semi-supervised exercise groups with different levels of exercise intensity (AIT and TCT), and a non-exercise control group (COU) (Ensenyat et al., 2017, 2020).
Participants
Initially, the study comprised 75 (26 men, 49 women) sedentary volunteers, 34 to 55 years old. To be eligible, participants should be between 30 and 55 years old, spent most of their awaken time in sedentary behaviour (sitting, lying down) and have at least one cardiometabolic risk factor (waist circumference >94.5 cm for men, and >89.5 cm for women; blood pressure ≥130/ 85 mmHg; triglycerides in plasma ≥150 mg/dL; HDLc in plasma <40 mg/dL for men and <50 mg/dL for women; fasting glucose ≥100 mg/dL). Individuals with severe health problems (morbid obesity, significant cardiopulmonary diseases, neuromuscular or psychiatric disorders) or diseases that may contraindicate performing physical exercise were excluded (Ensenyat et al., 2017, 2020).
Participants were recruited from primary healthcare centres and advertisements in the media. All participants were fully informed of the experimental procedures and provided written informed consent prior to enrollment. Then they were randomly assigned to one of the study groups (AIT, TCT, or COU) (Figure 1).
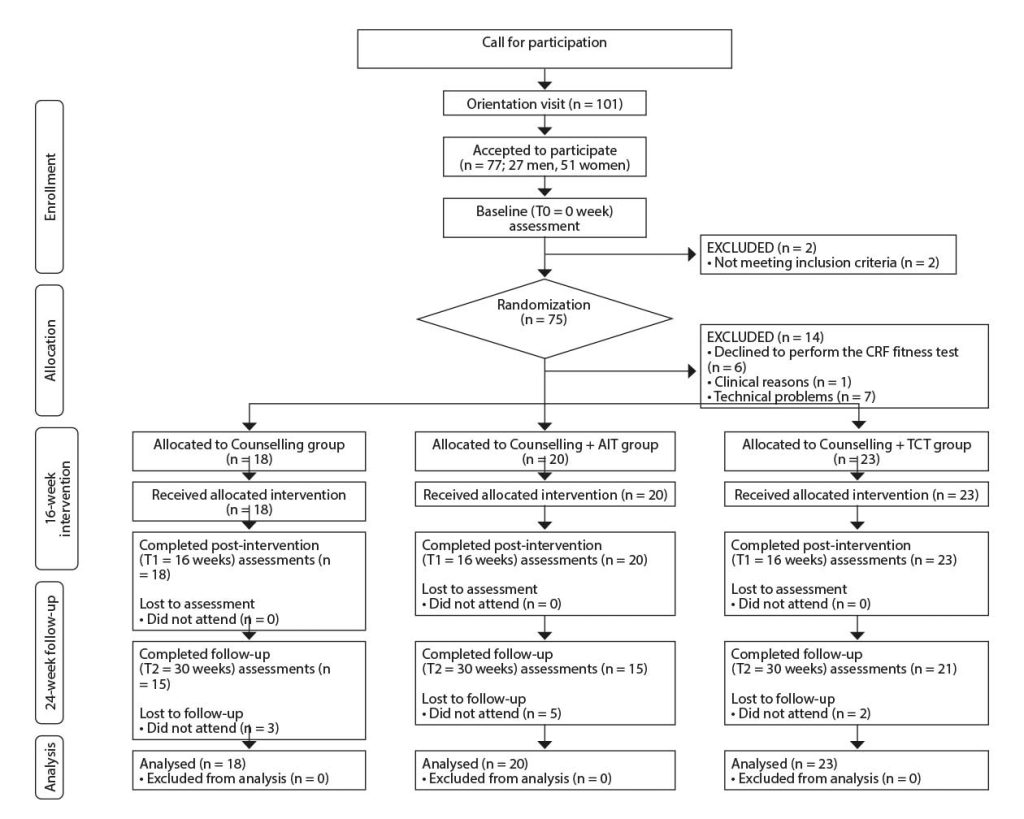
Note: Participants included in the Bellugat trial [Ensenyat, 2020 3078 /id] but that did not successfully perform the cardiorespiratory fitness test are excluded from the participant’s flow chart.
CRF: cardiorespiratory fitness test; AIT: aerobic interval training study group; COU: counselling study group. TCT: traditional continuous training study group.
T0: baseline assessment; T1: 16-week intervention assessment; T2: 24-week follow-up assessment.
Study procedures were approved by the Clinical Research Ethics Committee of the Institute for Primary Health Care Research Jordi Gol (IDIAP) (registration number: P15/122) and conformed to the Declaration of Helsinki (Cook et al., 2003). The study methods were consistent with the CONSORT guidelines (Schulz et al., 2011).
Intervention
The trial comprised a 16-week intervention and a 24-week follow-up. During the intervention phase, all groups were offered lifestyle counselling, which consisted of six 50-minute group sessions and four individual sessions lasting 40-50 minutes each (Ensenyat et al., 2017). The two exercise groups (AIT and TCT) also joined a semi-supervised physical exercise programme. This programme consisted of 16 supervised group training sessions lasting 50 minutes each and 32 self-administered sessions. The frequency of supervised sessions decreased throughout the intervention, while the frequency of the pre-scheduled self-administered sessions increased. All the training sessions comprised five parts: introduction, warm-up, main part (see below), cool-down, and conclusion. The main part differed between the AIT and TCT groups. AIT sessions consisted of four 4-minute repetitions at 80% of VO2peak, alternating with 2-minute active pauses at 60% of VO2peak and lasted a total of 24 minutes of effective exercise time while TCT sessions consisted of 40 minutes of moderate-intensity (60% of VO2peak) activities.
No discomfort or incidences were reported. Mean attendance of supervised exercise sessions, group and individual counselling sessions was 71.9% (SD = 20.2), 83.1% (SD = 17.1) and 98.8% (SD = 5.4) of the offered sessions, respectively. There were no differences between the study groups or genders.
Outcome measurements
Outcome measurements were recorded at baseline (T0 = week 0), after the intervention (T1 = week 16) and after the 24-week follow-up (T2 = week 40).
Cardiorespiratory graded exercise test
Participants completed a voluntary maximal graded exercise test on a cycle ergometer (Monark 828E, Monark, Sweden). After a two-minute warm-up stage at 10 W, the workload increased 20 W every two minutes until participants were unable to maintain the pre-established cadence (60 rpm) or if abnormal responses were observed (Balady et al., 2010).
During the test, ergoespirometric variables were measured using the Oxycon Mobile metabolic system (Oxycon Mobile, Carefusion, Germany). Gas calibrations were conducted before each test. Heart rate was measured using a Polar 610s chest heart rate monitor (Polar Electro YO, Kempele, Finland). Blood pressure was taken at 30s left within each stage using an automatic upper arm blood pressure monitor (Omron M, Omron Healthcare Europe B.V. Hoofddorp, the Netherlands). Before the end of the stage, participants reported their subjective perception of exercise using the Borg CR-10 scale (Borg & Kaijser, 2006). Chronotropy greater than 85% (Balady et al., 2010) and respiratory exchange ratio (RER) > 1.1 were used as criteria for having reached maximum exertion.
Data collection was undertaken in a well-ventilated laboratory room. Participants were instructed to fast and restrain from caffeine for 2 h prior the test and were asked to avoid any strenuous exercise the day before the test.
Maximal measures
Maximal (peak) ergoespirometric data for workload (W), oxygen uptake (VO2), dioxide production (VCO2), ventilation (VE), heart rate (HR), blood pressure (systolic blood pressure (SBP), diastolic blood pressure (DBP), and mean blood pressure (MBP)) were reported.
Effort-independent measures
To assess submaximal exercise capacity, the respiratory compensation point (RCP) was obtained by means of a concomitant increase in both ventilatory equivalents (VE/VO2 and VE/VCO2) and a decrease in partial pressure of CO2 (PETCO2) after a plateau (Beaver et al., 1986). Two observers independently calculated this point, and later corroborated it.
Ventilatory efficiency slope was obtained by linear regression analysis of the relationship between ventilation (VE) and carbon dioxide output (VCO2) with all exercise data (Arena et al., 2003). Oxygen uptake efficiency slope (OUES) was calculated with all exercise data according to Baba et al. (1996) by performing a linear regression analysis of the relationship between VO2 and the logarithm of VE (VO2 = alog10VE + b).
Cardiometabolic risk and lifestyle measures
A continuous metabolic syndrome severity score (MetSSS) was calculated, as described by Wiley and Carrington (2016). The score contains six risk factors: waist circumference (WC), triglyceride (TG) levels, high-density lipoprotein cholesterol (HDLc) levels, glycemia, systolic blood pressure (SBP) and diastolic blood pressure (DBP). WC was measured in triplicate following WHO guidelines (2011). Blood samples for determining plasma TG, HDLc and glucose levels were obtained after an overnight fast and analysed with automated methods at the university hospital. Blood pressure (BP) was measured at the level of the brachial artery of the dominant arm using an automated device (Omron M, Omron Healthcare Europe B.V. Hoofddorp, the Netherlands), with the participant in a relaxed sitting position.
Active and sedentary behaviour were assessed objectively using the ActiGraph GT3X+ accelerometer (ActiGraph LLC, Pensacola, FL, USA) over seven consecutive days. The data from the accelerometers were downloaded and analysed with the ActiLife 6.0 software (ActiGraph, Pensacola, FL, USA). Sleeping hours were excluded from the analysis. The data were analysed to yield an overall physical activity index (number of steps per day and as a vector magnitude (VM) in mean counts per minute (CPM)) and the percentage of time spent undertaking different levels of physical activity (Troiano et al., 2008).
A three-day 24-h dietary record (two weekdays and one weekend-day) was completed to monitor the dietary habits of each participant. The software PCN-CSNIS 1.0 was used to analyse the dietary records. The healthy eating index (HEI) was calculated to assess the participant’s dietary status (Basiotis et al., 2002).
Statistical methods
To determine the sample size for this study a power analysis was conducted using G*Power 3.1. (Faul et al., 2007) and the results obtained by Tjonna et al. (2008) in a supervised physical exercise intervention aimed at people with metabolic syndrome. To detect the expected changes with a bilateral hypothesis, an expected effect size of d = 0.46, a risk α of .05, a power of 80%, and repeated measures on 3 occasions and 3 study groups; the estimated number of participants was 48; 16 individuals per study group. The current sample of 62 people was considered sufficient to evaluate the hypotheses raised in relation to the CRF.
Data were checked for normality; then Chi-square (χ²) and Kruskal-Wallis (K-W) tests were used to compare the study arms at baseline (T0). Intention-to-treat analysis was performed for all the participants who completed the graded exercise test at baseline. Two-time frameworks were established: effectiveness (at the end of the intervention; T1) and persistence (at 24-week follow-up; T2). Wilcoxon and Kruskal-Wallis tests were used to analyse the differences between the study groups and changes over time. Continuous variables are expressed as the mean and standard deviation (SD). Categorical variables are expressed as counts (n) and percentages (%), unless otherwise specified.
Pearson (r) correlation was used to assess associations between the quantitative variables.
Significance was set at p < .05. For all analyses, SPSS 17.0 (SPSS, Chicago, IL, USA) was used.
Results
Participants
The baseline graded exercise test was completed successfully by 61 (23 men, 38 women) participants (aged 34 to 52 (M = 44.6, SD = 4.6) years) (Figure 1). There were no differences between the study groups regarding physical activity and dietary habits or any cardiometabolic risk factor at baseline.
Cardiometabolic risk and lifestyle outcomes
A significant time-effect was observed for MetSSS without group effect (Table 1). A significant small decrease in the MetSSS score was observed after the intervention. Changes persisted at follow-up, with 34.4% and 35.7% of the participants decreasing their MetSSS score by at least 0.5 points at T1 and T2. The effects were similar in all study groups.

Table 1
Metabolic syndrome risk score and lifestyle measures at baseline (T0), after the intervention (T1) and at 24-weeks follow-up period (T2)(n = 61).
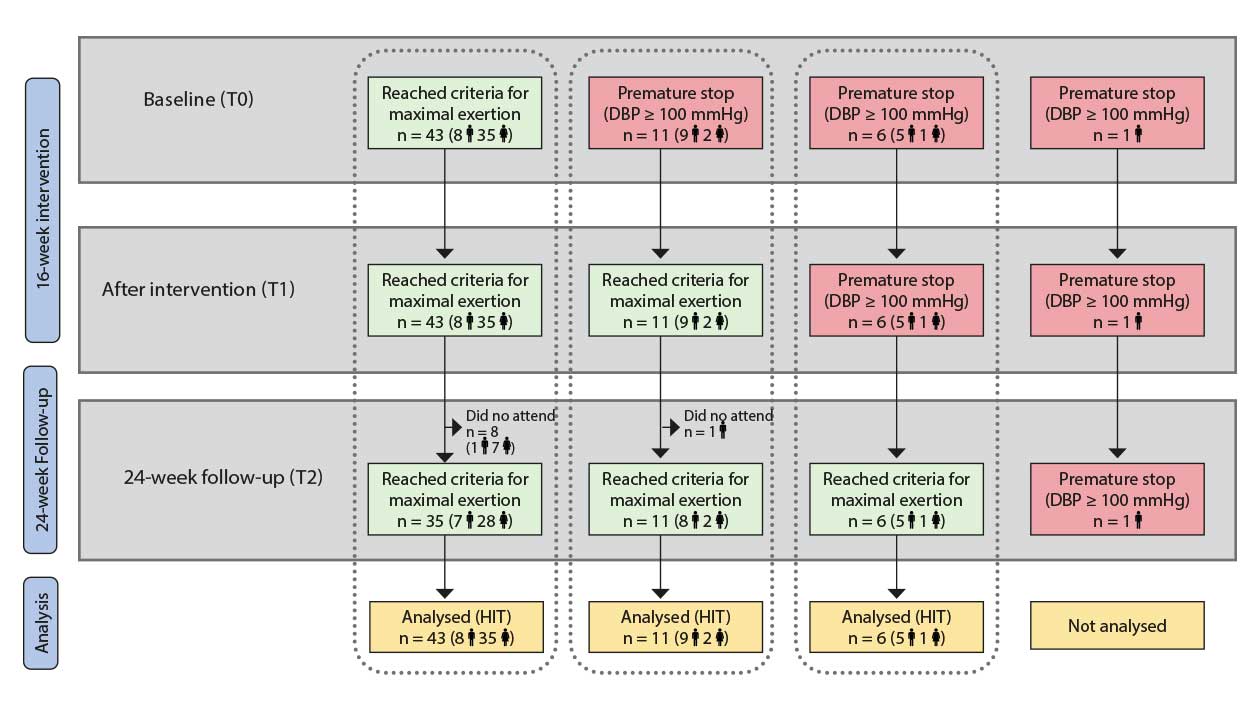
Note: Scenario A corresponds to participants who achieved maximal criteria for CRF test at T0, T1 and T2; Scenario B corresponds to participants who ended the CRF test due to high blood pressure response at T0 but achieved maximal criteria for CRF at T1 and T2; Scenario C corresponds to participants who ended the CRF test due to high blood pressure response at T0 and T1 but who achieved maximal criteria for CRF test at T2.
CRF: cardiorespiratory fitness; DBP: diastolic blood pressure; T0: baseline assessment; T1: after the 16-week intervention assessment; T2 at 24-week follow-up assessment.
Participants wore the accelerometer for 6.98 (SD = 0.6) days and 820.9 (SD = 77) minutes per day. A significant time-effect was observed for steps/day, overall activity, sedentary time, MVPA and HEI without group-effect (Table 1).
Cardiorespiratory graded exercise test
Sixty-one participants completed the baseline cardiorespiratory test assessment. Forty-three (70.5%) participants accomplished it satisfactorily and reached criteria for maximal exertion. As Figure 2 shows, these participants also reached maximal effort levels at T1 and T2 (Scenario A). The other 18 participants (29.5%) had to end the baseline test prematurely due to abnormal blood pressure response. (Scenario B and Scenario C). There were no differences between study groups regarding reasons to end the cardiorespiratory test (χ² = 1.431, p = .489).
We feel that maximal data cannot be analysed with all participants/scenarios pooled together. For that reason, we have split the analysis of VO2peak into three scenarios (Table 2). Effort-independent data have been analysed with all participants pooled together.

Table 2
Cardiorespiratory fitness test data at baseline (T0), after the intervention (T1) and at 24-weeks follow-up period (T2).
VO2peak
Scenario A: At baseline (T0), there were no significant differences between study groups in relation to peak values. Peak respiratory exchange ratio (RERpeak) (M = 1.19, SD = 0.07), chronotropy (M = 85.5%, SD = 17.7) and Borg CR-10 values (M = 7.8, SD = 1.8) suggest a high level of effort during the test.
A significant time-effect was observed for peak workload (Wpeak) without group effect (Table 3a). Despite this improvement, VO2peak did not change after intervention or at follow-up. Mean VO2peak increases tended to be better in the AIT group, but changes were lower than the clinical threshold of 3.5 mL/kg/min (Figure 3). However, the percentage of participants who improved their VO2peak above this threshold was higher in the AIT group (36%) than in the other groups (TCT = 28%; COU = 18%). At follow-up, the improvement persisted in 28% of AIT participants, but only in 5% and 18% of participants for TCT and COU.
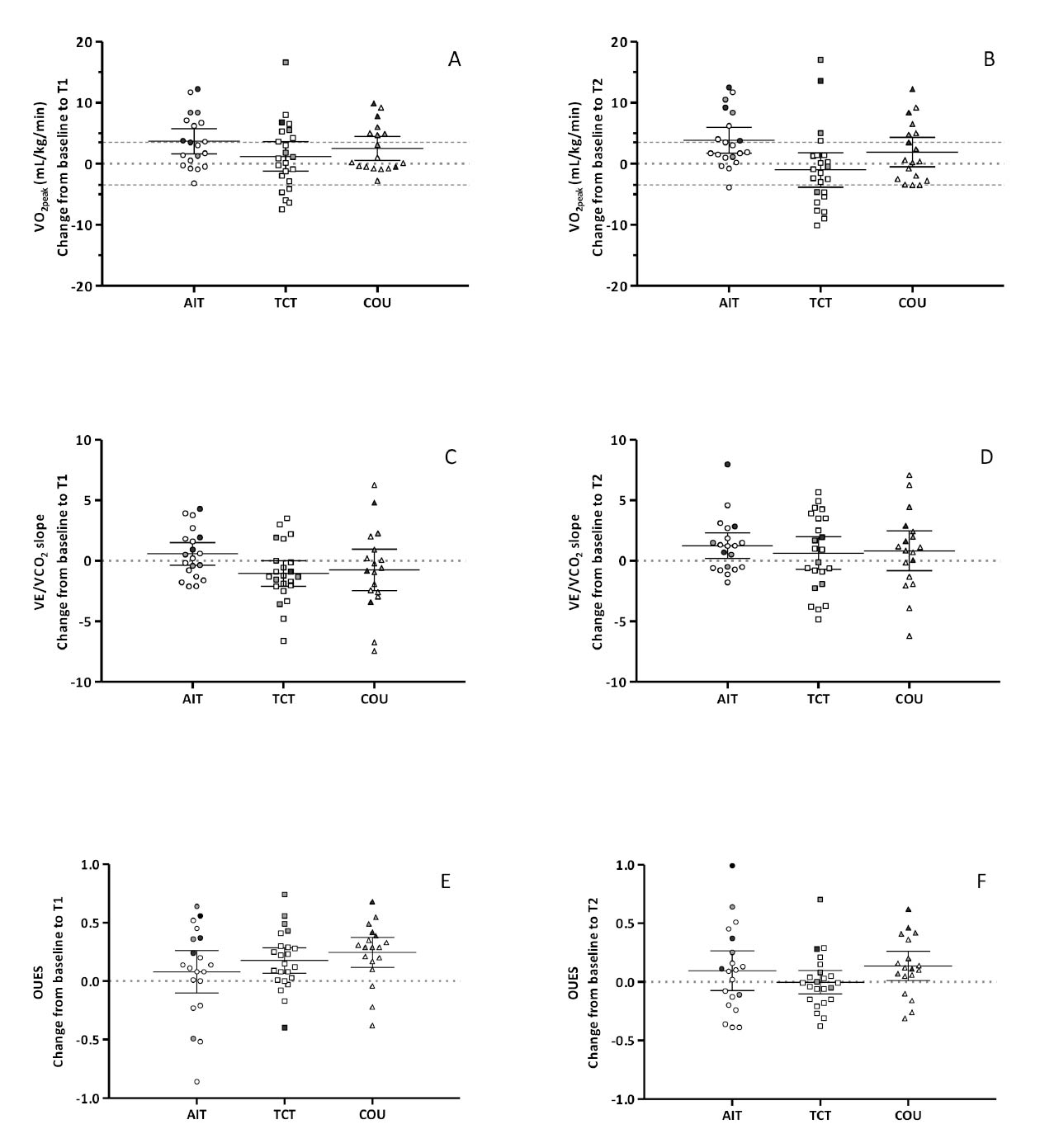
Note: T1: after the 16-week intervention assessment; T2 at 24-week follow-up assessment.
AIT: aerobic interval training study group; COU: counselling study group; TCT: traditional continuous training study group.
OUES: oxygen uptake efficiency slope; VCO2: carbon dioxide production; VE: ventilation; VO2: oxygen uptake;
VT: Ventilatory threshold; W: power output.
Each point indicates an individual participant.
Light symbols indicate Scenario A; Grey symbols indicate Scenario B, and Black grey symbols indicate Scenario C.

Table 3a
Cardiorespiratory fitness test data at baseline (T0), after the intervention (T1) and at 24-weeks follow-up period (T2) for Scenario A (n = 43).
Scenario B: As Figure 2 shows, eleven participants had to end the baseline test prematurely (RERpeak (M = 1.02, SD = 0.11), chronotropy (M = 60.8%, SD = 21.4), Borg CR-10 values (M = 5.3, SD = 2.0)). No statistically significant differences between the study groups were observed. At the end of the intervention these participants improved their adaptation to effort and reached maximal exertion as well as at follow-up. A moderate positive time-effect was observed for VO2peak without study group effects (Table 3b). Seven participants (63.6%) improved their VO2peak by at least 3.5 mL/kg/min at the end of the intervention and this remained elevated in six of them at follow-up.

Table 3b
Cardiorespiratory fitness test data at baseline (T0), after the intervention (T1) and at 24-weeks follow-up period (T2) for Scenario B (n = 11).
Scenario C: Six participants had to end the cardiorespiratory exercise test prematurely at baseline and at the end of the intervention but were able to reach maximal criteria at follow-up (RERpeak (M = 1.2, SD = 0.09), chronotropy (M = 94.6%, SD = 12.1), Borg CR-10 values (M = 7.3, SD = 1.7)).
Although at the end of the intervention (T1) these participants had to end the cardiorespiratory test prematurely, VO2peak showed a large significant increase. At follow-up, VO2peak improved further, with all 6 participants increasing VO2peak by more than 3.5 mL/kg/min (Table 3c).

Table 3c
Cardiorespiratory fitness test data at baseline (T0), after the intervention (T1) and at 24-weeks follow-up period (T2) for Scenario C (n = 6).
Effort independent data
Small but significant improvements were observed in the WRCP, VO2RCP and OUES at the end of the intervention without group effect. These increases did not persist at follow-up. However, considering only scenario B and C; WRCP, VO2RCP and OUES show large and persistent increases. No differences between study groups were appreciated. No time-effect was observed for VE-VCO2 slope.
Discussion and Conclusion
The present study evaluates the effectiveness of a 16-week intervention of semi-supervised interval training exercise programme alongside lifestyle counselling (AIT) on CRF in terms of VO2peak, VO2RCP, VE-VCO2 slope and OUES, in sedentary adults with cardiometabolic risk factors. The intervention was implemented in a real-world setting and compared to low-to-moderate-intensity continuous training plus lifestyle counselling (TCT) and to lifestyle counselling alone (COU).
AIT was well accepted by the participants with rather high levels of adherence to the exercise sessions. All study groups exhibited comparable modest decreases in MetSSS and positive lifestyle changes that persisted in the medium term.
All intervention programmes induced significant changes in CRF variables. Although the effects of the AIT intervention on CRF and physiological adaptation to exertion did not differ substantially from TCT or COU, the number of participants increasing their VO2peak was slightly higher. Changes in VO2peak differed according to whether or not the physiological adaptation to maximal graded exercise testing was satisfactory at baseline. Intervention was more effective for individuals with a poorer physiological adaptation to the graded exercise. All intervention programmes induced comparable significant non-persistent increases in VO2VT, no changes in VE-VCO2 slope and persistent improvements in OUES.
Several recent studies have shown that HIIT is effective in producing modest-to-moderate improvements in VO2peak/VO2max after 16-week (48 sessions) to 4-week (12 sessions) periods (Blackwell et al., 2017). Two previous meta-analyses reported greater effects of HIT compared to moderate-continuous training (Milanović et al., 2015; Pattyn et al., 2014).
These studies were mainly supervised and reported only the data from individuals who reached symptom-limited exercise at the CRF test. They reported increases in VO2peak ranging from 9-to-27% (Blackwell et al., 2017; Guio de Prada et al., 2019b). These values are greater than ours. However, the present study differs from the others in its semi-supervised nature and in the descending weekly frequency of supervised sessions. Moreover, as most studies include only individuals who completed at least 85% of the training sessions, it cannot be discarded that high values were due to the exclusion of non-responders from the analysis. Our data are consistent with Blackwell et al. (2017) who observed a lower improvement in VO2peak in the group that trained at home (≈ 10%) than in the group that received supervised exercise training (≈ 20%).
Although exercise intensity appears to be a critical factor for improving CRF, the baseline CRF level determines the individual exercise intensity needed to generate clinically significant increases. Low-fit individuals (CRF < 10 metabolic equivalent (METs)) will need a training intensity of 50% heart rate reserve (HRR) or VO2 reserve (VO2R), while a high-fit individual (CRF > 14 METs) will need a higher intensity (> 85% HRR or VO2R) (Ross et al., 2016). This could explain why participants who at baseline reached criteria for maximal effort did not improve their VO2peak. In these participants, the overall training load may have been too low to induce large changes in VO2peak in any study group. Nonetheless, the fact that the number of participants in AIT who improved their VO2peak was higher than in TCT and COU seems to support the hypothesis that even a semi-supervised AIT may be more effective than TCT and COU.
In contrast, for participants who presented a maladaptation to graded exertion at baseline, it is possible that the training load or even the lifestyle change were enough to induce a better response to exertion. Thus, in that case, large improvements in VO2peak were recorded in all study groups. This is consistent with findings in Milanović’s meta-analysis (Milanović et al., 2015) that revealed a likely moderate greater beneficial improvement in VO2max for participants with lower baseline CRF (for both HIT and endurance training).
Low cardiorespiratory fitness (VO2peak) is an independent and strong predictor of premature mortality (Lee et al., 2010) that has been associated with a greater risk of metabolic syndrome (Lee, 2020) thus, even small improvements of CRF can have positive effects on health.
Most daily life activities do not require maximal effort; thus, it has been proposed that indicators of submaximal exercise capacity may be of interest from a health point of view (Balady et al., 2010). Ventilatory thresholds (VT and RCP) are widely used indices of submaximal exercise capacity. In the field of exercise-and-health, several studies report increases in the workload and the VO2 achieved at the first ventilatory threshold (VT) in sedentary individuals (Blackwell et al., 2017) or in patients with metabolic syndrome (Guio de Prada et al., 2019a) or with CHF (Kemps et al., 2010). To our knowledge, few studies have analysed the effects of training on the second ventilatory threshold (RCP). Guio de Prada et al. (2019b) reported an increase in VT and in RCP after HIIT in 76 sedentary individuals with obesity. Our results confirm that RCP is also sensitive to the effects of semi-supervised training but changes are not persistent.
VE-VCO2 slope reflects the ventilation for a given VCO2 production (Akkerman et al., 2010) and has been considered as an indicator of CRF and a predictor of mortality in patients with chronic heart failure (CHF). In the current study, none of the three intervention programmes had an impact on VE-VCO2 slope. Our data agree with other studies that have observed no changes in VE-VCO2 slope after exercise training in patients with cardiovascular diseases (Kemps et al., 2010; Nilsson et al., 2019; Pattyn et al., 2014) but contrast with others that reported decreases of VE-VCO2 slope in individuals with high values (>35) at baseline (Van Laethem et al., 2007). Thus, the possible training-induced changes might be more limited in participants with slope values within the normal range of 20–30. Still, Anaya (Anaya et al., 2009) has reported a significant dose-dependent trend for changes of VE-VCO2 slope in post-menopausal women.
In the current study, the lack of changes in VE-VCO2 slope could be attributed to a training load too low to induce changes in the VE-VCO2 slope or alternatively, it could be because the VE-VCO2 slope is not sensitive enough to the effects of exercise training in sedentary individuals with slope values lower that 30.
OUES is considered an integrated measurement that reflects the coordination of pulmonary and cardiovascular systems and the extraction of O2 during exercise (Balady et al., 2010). Our data show that all intervention programmes induced comparable significant improvement in OUES. It seems that OUES may be more sensitive to change than VE-VCO2 slope. Data are consistent with other studies that have reported similar improvements in OUES after exercise training interventions in patients with cardiometabolic diseases (Kemps et al., 2010; Nilsson et al., 2019). However, in another study (Mourot et al., 2004), where participants were healthy, physically active young women, 18 sessions of intermittent endurance training had no effects on OUES or on VE-VCO2 slope, despite significant increases in VO2peak and VO2VT.
The semi-supervised nature of the exercise training could be considered a limitation. In this study, the participants were closely supervised by a physical activity professional at the beginning of the programme but should became more self-governing with time. This may explain the lack of greater effects of the exercise training programmes on cardiorespiratory fitness (VO2peak, VO2RCP, VE-VCO2 slope, OUES) compared with the COU group or other studies. We are aware that we did not adequately track the participants during the self-administered sessions. Non-compliance with the expected training load could result in lower training stimuli and hence with lower adaptations. For future research, we recommend increasing the number of supervised training sessions and strengthening the monitoring of self-administered sessions with strategies, such as e-based communication platforms.
A major strength of this study was that the 16-week intervention combined the semi-supervised exercise training programme with lifestyle counselling to promote behavioural change. This study is unique as, unlike other AIT studies, it not only considers physical exercise, but also links it to strategies that promote behavioural change and empowerment, and assesses the medium-term persistence of the effects. Most AIT interventions have been performed in a highly controlled experimental setting for a limited time period and report only immediate effects of the interventions. In the current study diverse beneficial changes (CRF and lifestyle), in particular in less fit individuals (VO2peak, OUES), persisted at 24-week follow-up.
In conclusion the study demonstrates that a 16-week intervention of semi-supervised AIT alongside lifestyle counselling could be (1) a feasible strategy to be translated into the real-word exercise programme aimed at sedentary individuals with cardiometabolic risk factors; and was (2) equally effective to TCT or COU programmes in increasing VO2peak in low-fit individuals, although the number of responders was higher in AIT than in TCT or COU study groups; and (3) as effective as TCT or COU in improving effort-independent variables such as VO2RCP, and OUES. However, (4) none of the three intervention programmes had an impact on VE-VCO2 slope.
References
[1] Akkerman, M., Van Brussel, M., Hulzebos, E., Vanhees, L., Helders, P. J. M., & Takken, T. (2010). The Oxygen Uptake Efficiency Slope. Journal of Cardiopulmonary Rehabilitation and Prevention, 30(6), 357–373. https://doi.org/10.1097/HCR.0b013e3181ebf316
[2] Anaya, S. A., Church, T. S., Blair, S. N., Myers, J. N., & Earnest, C. P. (2009). Exercise dose-response of the V̇ E/V̇CO 2 slope in postmenopausal women in the DREW study. Medicine and Science in Sports and Exercise, 41(5), 971–976. https://doi.org/10.1249/MSS.0b013e3181930009
[3] Arena, R., Myers, J., Aslam, S. S., Varughese, E. B., & Peberdy, M. A. (2003). Technical considerations related to the minute ventilation/carbon dioxide output slope in patients with heart failure. Chest, 124(2), 720–727. https://doi.org/10.1378/chest.124.2.720
[4] Baba, R., Nagashima, M., Goto, M., Nagano, Y., Yokota, M., Tauchi, N., & Nishibata, K. (1996). Oxygen uptake efficiency slope: A new index of cardiorespiratory functional reserve derived from the relation between oxygen uptake and minute ventilation during incremental exercise. Journal of the American College of Cardiology, 28(6), 1567–1572. https://doi.org/10.1016/S0735-1097(96)00412-3
[5] Balady, G. J., Arena, R., Sietsema, K., Myers, J., Coke, L., Fletcher, G. F., Forman, D., Franklin, B., Guazzi, M., Gulati, M., Keteyian, S. J., Lavie, C. J., Macko, R., & Mancini, D. (2010). Clinician’s Guide to Cardiopulmonary Exercise Testing in Adults A Scientific Statement From the American Heart Association. 191–225. https://doi.org/10.1161/CIR.0b013e3181e52e69
[6] Basiotis, P., Carlson, A., Gerrior, S., Juan, W., & Lino, M. (2002). The Healthy Eating Index 1999-2000. Washington, DC: US Department of Agriculture, Center for Nutrition Policy and Promotion. https://www.usda.gov
[7] Beaver, W. L., Wasserman, K., & Whipp, B. J. (1986). A new method for detecting anaerobic threshold by gas exchange. Journal of Applied Physiology, 60(6), 2020–2027. https://doi.org/10.1152/jappl.1986.60.6.2020
[8] Blackwell, J., Atherton, P. J., Smith, K., Doleman, B., Williams, J. P., Lund, J. N., & Phillips, B. E. (2017). The efficacy of unsupervised home-based exercise regimens in comparison to supervised laboratory-based exercise training upon cardio-respiratory health facets. Physiological Reports, 5(17). https://doi.org/10.14814/phy2.13390
[9] Borg, E., & Kaijser, L. (2006). A comparison between three rating scales for perceived exertion and two different work tests. Scandinavian Journal of Medicine and Science in Sports, 16(1), 57–69. https://doi.org/10.1111/j.1600-0838.2005.00448.x
[10] Cook, R. J., Dickens, B. M., & Fathalla, M. F. (2003). World Medical Association Declaration of Helsinki: Ethical Principles for Medical Research Involving Human Subjects. In Reproductive Health and Human Rights (Vol. 81, Issue 3, pp. 428–432). Oxford: Oxford University Press. https://doi.org/10.1093/acprof:oso/9780199241323.003.0025
[11] Ensenyat, A., Espigares-Tribo, G., Machado-Da-Silva, L., Sinfreu-Bergués, X., & Blanco, A. (2020). Semisupervised physical exercise and lifestyle counseling in cardiometabolic risk management in sedentary adults: Controlled randomized trial (BELLUGAT). Journal of Physical Activity and Health, 17(7), 744–755. https://doi.org/10.1123/jpah.2019-0409
[12] Ensenyat, A., Espigares-Tribo, G., Machado, L., Verdejo, F. J., Rodriguez-Arregui, R., Serrano, J., Miret, M., Galindo, G., Blanco, A., Marsal, J.-R., Sarriegui, S., Sinfreu-Bergues, X., & Serra-Paya, N. (2017). Metabolic risk management, physical exercise and lifestyle counselling in low-active adults: controlled randomized trial (BELLUGAT). BMC Public Health, 17(1), 257. https://doi.org/10.1186/s12889-017-4144-8
[13] Faul, F., Erdfelder, E., Lang, A.-G., & Buchner, A. (2007). G*Power 3: A flexible statistical power analysis program for the social, behavioral, and biomedical sciences. Behavior Research Methods, 39(2), 175–191. https://doi.org/10.3758/BF03193146
[14] Gami, A. S., Witt, B. J., Howard, D. E., Erwin, P. J., Gami, L. A., Somers, V. K., & Montori, V. M. (2007). Metabolic Syndrome and Risk of Incident Cardiovascular Events and Death. A Systematic Review and Meta-Analysis of Longitudinal Studies. Journal of the American College of Cardiology, 49(4), 403–414. https://doi.org/10.1016/j.jacc.2006.09.032
[15] Guio de Prada, V., Ortega, J. F., Morales-Palomo, F., Ramirez-Jimenez, M., Moreno-Cabañas, A., & Mora-Rodriguez, R. (2019a). Women with metabolic syndrome show similar health benefits from high-intensity interval training than men. PloS One, 14(12), e0225893. https://doi.org/10.1371/journal.pone.0225893
[16] Guio de Prada, V., Ortega, J. F., Ramirez-Jimenez, M., Morales-Palomo, F., Pallares, J. G., & Mora-Rodriguez, R. (2019b). Training intensity relative to ventilatory thresholds determines cardiorespiratory fitness improvements in sedentary adults with obesity. European Journal of Sport Science, 19(4), 549-556. https://doi.org/10.1080/17461391.2018.1540659
[17] Kemps, H. M. C., De Vries, W. R., Schmikli, S. L., Zonderland, M. L., Hoogeveen, A. R., Thijssen, E. J. M., & Schep, G. (2010). Assessment of the effects of physical training in patients with chronic heart failure: The utility of effort-independent exercise variables. European Journal of Applied Physiology, 108(3), 469–476. https://doi.org/10.1007/s00421-009-1230-3
[18] Knaeps, S., Bourgois, J. G., Charlier, R., Mertens, E., Lefevre, J., & Wijndaele, K. (2018). Ten-year change in sedentary behaviour, moderate-to-vigorous physical activity, cardiorespiratory fitness and cardiometabolic risk: independent associations and mediation analysis. British Journal of Sports Medicine, 52(16), 1063–1068. https://doi.org/10.1136/bjsports-2016-096083
[19] Lee, D., Artero, E. G., Xuemei Sui, & Blair, S. N. (2010). Review: Mortality trends in the general population: the importance of cardiorespiratory fitness. Journal of Psychopharmacology, 24(4_suppl), 27–35. https://doi.org/10.1177/1359786810382057
[20] Lee, J. (2020). Influences of Cardiovascular Fitness and Body Fatness on the Risk of Metabolic Syndrome: A Systematic Review and Meta-Analysis. American Journal of Health Promotion, 34(7), 796–805. https://doi.org/10.1177/0890117120925347
[21] Milanović, Z., Sporiš, G., & Weston, M. (2015). Effectiveness of High-Intensity Interval Training (HIT) and Continuous Endurance Training for VO2max Improvements: A Systematic Review and Meta-Analysis of Controlled Trials. Sports Medicine, 45(10), 1469–1481. https://doi.org/10.1007/s40279-015-0365-0
[22] Mourot, L., Perrey, S., Tordi, N., & Rouillon, J. D. (2004). Evaluation of Fitness Level by the Oxygen Uptake Efficiency Slope After a Short-Term Intermittent Endurance Training. International Journal of Sports Medicine, 25(2), 85–91. https://doi.org/10.1055/s-2004-819943
[23] Myers, J., McAuley, P., Lavie, C. J., Despres, J.-P., Arena, R., & Kokkinos, P. (2015). Physical Activity and Cardiorespiratory Fitness as Major Markers of Cardiovascular Risk: Their Independent and Interwoven Importance to Health Status. Progress in Cardiovascular Diseases, 57(4), 306–314. https://doi.org/10.1016/j.pcad.2014.09.011
[24] Nilsson, H., Nylander, E., Borg, S., Tamás, É., & Hedman, K. (2019). Cardiopulmonary exercise testing for evaluation of a randomized exercise training intervention following aortic valve replacement. Clinical Physiology and Functional Imaging, 39(1), 103–110. https://doi.org/10.1111/cpf.12545
[25] Pattyn, N., Coeckelberghs, E., Buys, R., Cornelissen, V. A., & Vanhees, L. (2014). Aerobic Interval Training vs. Moderate Continuous Training in Coronary Artery Disease Patients: A Systematic Review and Meta-Analysis. Sports Medicine, 44(5), 687–700. https://doi.org/10.1007/s40279-014-0158-x
[26] Pattyn, N., Cornelissen, V. A., Eshghi, S. R. T., & Vanhees, L. (2013). The effect of exercise on the cardiovascular risk factors constituting the metabolic syndrome: A meta-analysis of controlled trials. Sports Medicine, 43(2), 121–133. https://doi.org/10.1007/s40279-012-0003-z
[27] Pedersen, B. K., & Saltin, B. (2015). Exercise as medicine - evidence for prescribing exercise as therapy in 26 different chronic diseases. Scandinavian Journal of Medicine & Science in Sports, 25, 1–72. https://doi.org/10.1111/sms.12581
[28] Ross, R., Blair, S. N., Arena, R., Church, T. S., Després, J.-P., Franklin, B. A., Haskell, W. L., Kaminsky, L. A., Levine, B. D., Lavie, C. J., Myers, J., Niebauer, J., Sallis, R., Sawada, S. S., Sui, X., & Wisløff, U. (2016). Importance of Assessing Cardiorespiratory Fitness in Clinical Practice: A Case for Fitness as a Clinical Vital Sign: A Scientific Statement From the American Heart Association. Circulation, 134(24). https://doi.org/10.1161/CIR.0000000000000461
[29] Schulz, Altman, D. G., & Moher, D. (2011). CONSORT 2010 statement: Updated guidelines for reporting parallel group randomised trials. International Journal of Surgery, 9(8), 672–677. https://doi.org/10.1016/j.ijsu.2011.09.004
[30] Tjønna, A. E., Lee, S. J., Rognmo, Ø., Stølen, T. O., Bye, A., Haram, P. M., Loennechen, J. P., Al-Share, Q. Y., Skogvoll, E., Slørdahl, S. A., Kemi, O. J., Najjar, S. M., & Wisløff, U. (2008). Aerobic Interval Training Versus Continuous Moderate Exercise as a Treatment for the Metabolic Syndrome. Circulation, 118(4), 346–354. https://doi.org/10.1161/CIRCULATIONAHA.108.772822
[31] Tjønna, A. E., Ramos, J. S., Pressler, A., Halle, M., Jungbluth, K., Ermacora, E., Salvesen, Ø., Rodrigues, J., Bueno, C. R., Munk, P. S., Coombes, J., & Wisløff, U. (2018). EX-MET study: exercise in prevention on of metabolic syndrome – a randomized multicenter trial: rational and design. BMC Public Health, 18(1), 437. https://doi.org/10.1186/s12889-018-5343-7
[32] Troiano, R. P., Berrigan, D., Dodd, K. W., Mâsse, L. C., Tilert, T., & Mcdowell, M. (2008). Physical Activity in the United States Measured by Accelerometer. Medicine & Science in Sports & Exercise, 40(1), 181–188. https://doi.org/10.1249/mss.0b013e31815a51b3
[33] Van Laethem, C., Van De Veire, N., De Backer, G., Bihija, S., Seghers, T., Cambier, D., Vanderheyden, M., & De Sutter, J. (2007). Response of the oxygen uptake efficiency slope to exercise training in patients with chronic heart failure. European Journal of Heart Failure, 9(6–7), 625–629. https://doi.org/10.1016/j.ejheart.2007.01.007
[34] WHO. (2018). Healthy-lifestyle counselling. In HEARTS Technical package for cardiovascular disease management in primary health care. https://apps.who.int/iris/bitstream/handle/10665/260422/WHO-NMH-NVI-18.1-eng.pdf?sequence=1
[35] Wiley, J. F., & Carrington, M. J. (2016). A metabolic syndrome severity score: A tool to quantify cardio-metabolic risk factors. Preventive Medicine, 88, 189–195. https://doi.org/10.1016/J.YPMED.2016.04.006
[36] Wisløff, U., Støylen, A., Loennechen, J. P., Bruvold, M., Rognmo, Ø., Haram, P. M., Tjønna, A. E., Helgerud, J., Slørdahl, S. A., Lee, S. J., Videm, V., Bye, A., Smith, G. L., Najjar, S. M., Ellingsen, Ø., & Skjærpe, T. (2007). Superior cardiovascular effect of aerobic interval training versus moderate continuous training in heart failure patients: A randomized study. Circulation, 115(24), 3086–3094. https://doi.org/10.1161/CIRCULATIONAHA.106.675041
[37] World Health Organization. (2011). Waist Circumference and Waist–Hip Ratio: Report of a WHO Expert Consultation: Ginebra, 8-11, 2011. 1, 8–11. www.who.int
ISSN: 2014-0983
Received: September 8, 2022
Accepted: February 22, 2023
Published: October 1, 2023
Editor: © Generalitat de Catalunya Departament de la Presidència Institut Nacional d’Educació Física de Catalunya (INEFC)
© Copyright Generalitat de Catalunya (INEFC). This article is available from url https://www.revista-apunts.com/. This work is licensed under a Creative Commons Attribution-NonCommercial-NoDerivatives 4.0 International License. The images or other third party material in this article are included in the article’s Creative Commons license, unless indicated otherwise in the credit line; if the material is not included under the Creative Commons license, users will need to obtain permission from the license holder to reproduce the material. To view a copy of this license, visit https://creativecommons.org/licenses/by-nc-nd/4.0/deed.en