Changes in Front Crawl Technique Caused by Different Technical Exercises
*Corresponding author: Francisco Cuenca-Fernández cuenca@ugr.es
Cite this article
López-Contreras, G., Campaña-Montilla, M. P., Cuenca-Fernández, F., & Arellano, R. (2022). Changes in Front Crawl Technique Caused by Different Technical Exercises. Apunts Educación Física y Deportes, 148, 34-41. https://doi.org/10.5672/apunts.2014-0983.es.(2022/2).148.05
Abstract
Some swimming exercises alter the rotation around the longitudinal axis of the body. This study determined the differences in, and the symmetry of, shoulder and hip rotation caused by different exercises. In a cross-sectional design, 16 trained swimmers performed six different freestyle swimming protocols: i) breathing holding (BH); ii) breathing right (BR); iii) arm extended breathing right (AEBR) or iv) breathing left (AEBL); v) arm close breathing right (ACBR) or vi) left (ACBL). The exercises were recorded on video using two frontal cameras and the angles were measured by Kinovea®. Two-way ANOVA was used to explore protocol-exercise interaction. Differences between the executing arm (AE) side and non-executing arm (NEA) side were compared using paired-sample t-tests. The shoulders rotated more than the hips and reached their maximum rotation earlier. Breathing increased rotation by ~7-12˚. ACBR and ACBL showed lower rotation ranges than the other protocols, but produced significant asymmetries between the executing arm side (shoulder: 8-19˚; hip: 12-17˚) and non-executing arm side (shoulder: 66-77˚; hip: 51-68˚). ACBR and ACBL showed similar rotational ranges to normal swimming, but had a different stroke cycle time (1.5 s vs. 1.3 s). The changes between the rotation of shoulders and hips were not uniform and were especially favoured by swimming exercises, especially when breathing. Coaches should reconsider including these exercises in swimming programs.
Introduction
Front crawl swimming often presents persistent problems while it is learnt, such as difficulty breathing and lack of rhythm or coordination (Zhang, 2014). For this reason, specific exercises (also called drills) to teach and correct swimming techniques are applied (Bielec et al., 2008; Lucero, 2015; Wen et al., 2016). Some swimming drills require excessive skill and cognitive involvement and therefore can be difficult for beginners, however, others, such as breath-hold swimming drills and one-arm front crawl are commonly used to reproduce certain competition conditions or as a procedure to force the swimmer to focus more on a specific aspect of their technique (Zhang, 2014; Lucero, 2015).
Although the scientific literature has not produced a detailed study on this topic, there is evidence based on studies focused on changes in the longitudinal rotation of the body during swimming (i.e., the roll). Basically, this rotation increases the effectiveness of the arm pull, since it allows the use of large muscle groups (Prichard, 1993; Andersen et al., 2020). Breathing and arm recovery are also smoother, as the head and shoulders are given more room to move as the body rotates (Payton et al., 1997; Psycharakis & Sanders, 2010). However, some studies have shown that arm trajectories can be modified and negatively affected by excessive body rotation (Payton et al., 1997; Psycharakis & Sanders, 2010; Lecrivain et al., 2010). Furthermore, since these changes are modulated by swimming speed (Psycharakis & Sanders, 2010), increasing upper body actions would reduce the overall range and duration of that rotation (Andersen et al., 2020; Yanai, 2001; Sanders & Psycharakis, 2009), thus restricting the turning effect in non-propulsive directions and keeping the body more hydrodynamic (Cappaert et al., 1995; Yanai, 2003). In fact, a common error found in the pool is the tendency to include these practices during low intensity sessions or as recovery between high intensity series, since this leads to a reduction in swimming rhythm and a transfer to actual competitive swimming. Therefore, regardless of the changes in technique, swimming exercises performed at low speeds would also result in increased rotation of the shoulders and hips.
Some authors have shown that, in swimmers, it is normal to find an asymmetry in the rotation of the body between the breathing side and the non-breathing side (Psycharakis & Sanders, 2010; Psycharakis & McCabe, 2011; de Souza-Castro et al ., 2007; Payton et al., 1999). However, the asymmetry caused by the actions of breathing can increase during swimming exercises if the swimmer is forced into a position that creates additional asymmetry per se. For example, single-arm front crawl exercises are common in swimming practices to improve breath coordination, to work more on one arm, or as a way to modify the swimmer’s technique (Lucero, 2015; Yanai and Hay, 2000; López-Contreras & Arellano, 2002). However, it is important to point out that the loss of propulsion and body stability that comes from restricting the actions of the non-executing arm (NEA) would produce a compensatory increase in the actions of the executing arm (EA), which could be related to a loss of stroke length (Cuenca-Fernández et al., 2020a). Therefore, little or no benefit may be obtained from these swimming exercises, especially if a consistent stroke rate is not maintained during the practice (Funai et al., 2019).
Lastly, although the shoulders have been shown to rotate more than the hips regardless of swimming speed (Andersen et al., 2020; Cappaert et al., 1995; Yanai, 2003), hip rotation increases during swimming trials as swimmers’ fatigue progresses, possibly due to less compensatory action from the legs (Psycharakis & Sanders, 2008). This suggests that a swimmer’s stability in the water seems to be affected by aspects of a multifactorial nature such as those caused by the breathing and kicking actions, as well as those caused by the slow swimming speed (Psycharakis & Sanders, 2010; Sanders & Psycharakis, 2009). Therefore, there is no reason to ignore the possible alterations that swimming exercises can produce.
At this point, and given that the intrinsic objective of a drill is to cause changes in actual swimming after continuous repetitive practice, we set out to see if those exercises performed at maximum speed would actually improve or worsen the swimming technique before incorporating them into a long-term training programme. Therefore, the purpose of this study was to determine the biomechanical differences between various common front crawl swimming exercises on the shoulder and hip rotation and their symmetry between the executing and non-executing arm (EA; NEA) sides. Our hypothesis was that certain swimming exercises would cause potentially critical alterations in technique.
Methodology
Experimental design
A cross-sectional design was used to explore swimming technique during six different front crawl protocols. The protocols consisted of: i) breathing holding (BH); ii) breathing right (BR); non-executing arm (NEA) extended breathing on the side of the executing arm (EA): iii) to the right (AEBR) and iv) to the left (AEBL); NEA close breathing on that side: v) to the right (ACBR) and vi) to the left (ACBL) (Figure 1). The six protocols were applied in a randomised fashion and differences in shoulder and hip rotation were observed among the participants.
Participants
A group of 16 national competitive swimmers (men n = 10; women n = 6), aged between 14 and 18 years, were informed of the possible risks and requirements of the test, and provided signed informed consent to participate in the experiment. Those under the age of 18 also provided parental consent. The inclusion criteria were: i) having the front crawl as their primary competition stroke; ii) breathing on the right side to maintain equality between the dominant side and the laterality of our subjects (21); and iii) have at least 5 years of regulated training. The study was designed in accordance with the Declaration of Helsinki for research on humans and was approved by the ethics committee of the University of Granada (code 852).
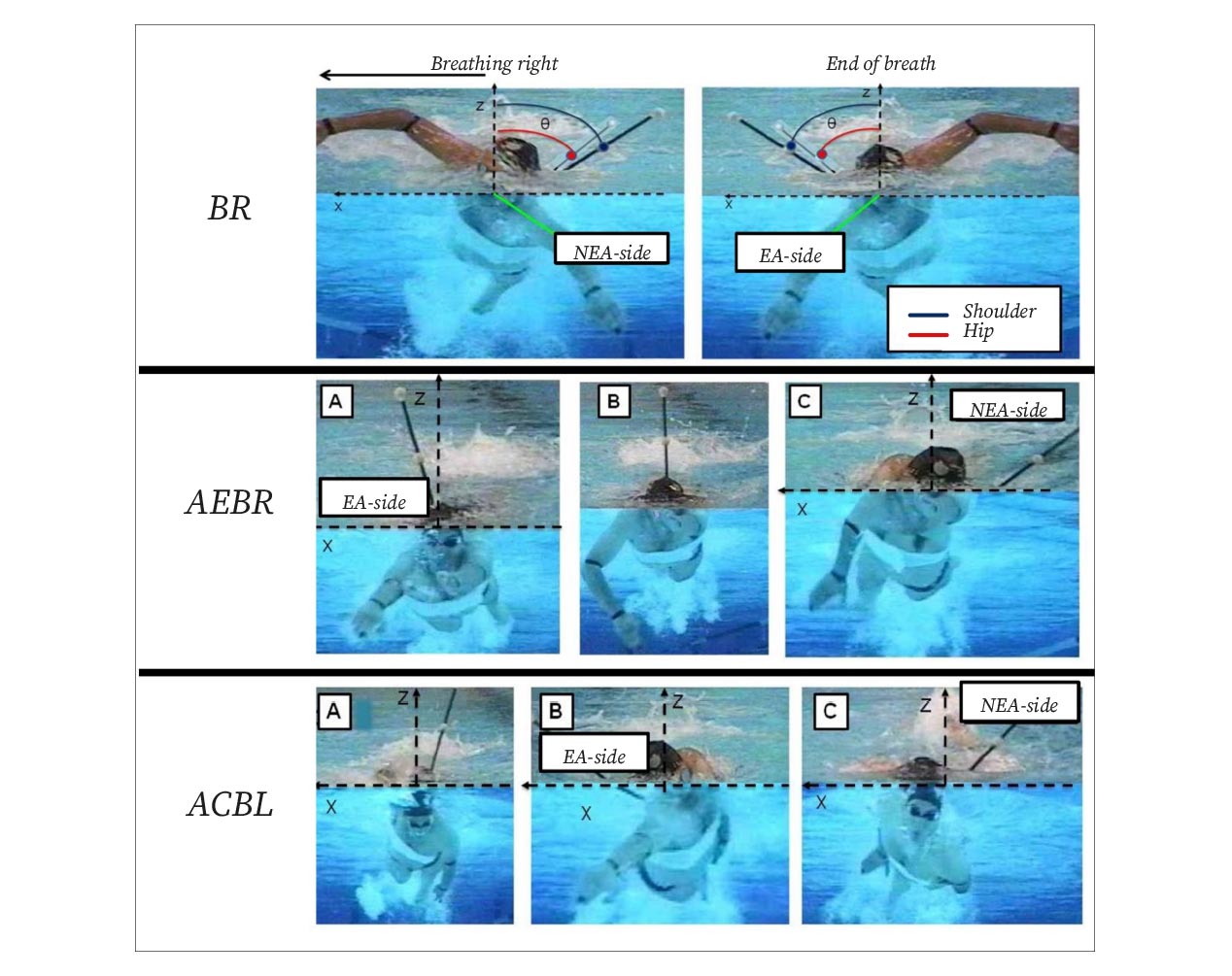
Breathing right (BR); extended arm extended breathing right (AEBR); arm close breathing left (ACBL); executing arm side (EA); non-executing arm side (NEA).
Procedure: materials and instruments
Before the test, the swimmers attended a familiarisation session to practice the swimming exercises and the evaluation process. The experimental context was a 25 x 12.5 m pool (water temperature = 27 ºC). The swimmers arrived at the pool within their usual afternoon training hours, having refrained from strenuous exercise for the previous 24 hours. On arrival, the elbow and wrist joints and the tip of the index finger were marked with black tape, and a warm-up consisting of 8 x 25 m front crawl swims before the start of the test. Each test protocol consisted of six 15-metre exercises and swimmers were asked to avoid swimming underwater.
Two dorsal acrylic bars were placed (length and width: 50 x 1 cm) on the swimmers through a base attached to a tightly fitting belt around the lower edges of the scapula and between the iliac crests (i.e., the lumbar region) (Figure 1). The black tape markings on the joints allow for precise digitisation (Andersen et al., 2020), and the bars are firmly attached to the body and do not slip (Payton et al., 1997; Liu et al., 1993). Once the bars were in place, the subjects were photographed lying in a ventral position in front of a reference fixed to the wall. This allowed calibration and ensured the correct positioning of the bar in a vertical position. The values for the maximum rotation of the shoulder and hip around the longitudinal axis of the body were measured by the position of the bars relative to the vertical position (in degrees º) (Figure 1).
Measured variables
The maximum rotation angles achieved on the EA side were measured during the first half of the total arm action, while the NEA side values were measured during breathing on the EA side (with the exception of ACBR and ACBL, because the breathing was on the NEA side). Two-dimensional (2D) photogrammetric techniques were used with two video cameras (S-VHS NV-MS4, 50 Hz, Panasonic Corp., Japan), located in the frontal plane (one of them on the surface and the other underwater), and the records were superimposed in space and time by means of a video switcher (Digital video switcher SE -900, Whittier, USA ). Each swimmer performed the exercises through an area that had been previously calibrated using a reference system (1.50 m long, 0.88 m wide and 0.93 meters high). This system, containing 15 landmarks of known locations, was placed in the centre of the lane used, and the established registration area ranged from 5 to 6.50 m from the front camera. The digitisation and the measurement of the angles were carried out with version 0.7.10 of Kinovea®.
Analysis of data
Descriptive statistics were expressed as mean ± SD (standard deviation) and confidence intervals (CI 95%). The effect sizes (d) of the differences obtained (small if 0 ≤ |d| ≤ .5; medium if .5 < |d| ≤ .8; and large if |d| > .8) (Andersen et al., 2020). The normality of the distribution was analysed using the Kolmogorov-Smirnov method. The differences between the “protocol” factor and the “shoulder vs. hip” were analysed using repeated measures two-way ANOVA and the subsequent post hoc tests were carried out using the Bonferroni method. Paired sample t-tests were performed to compare EA and NEA side shoulder and hip values under all conditions. Statistical significance was set at p < .05 and all statistical procedures were performed using the SPSS 23.0 program (IBM, Chicago, IL, USA). Test-retest (intraclass correlation coefficient [ICC]), intra- and inter-observer reliability was used to assess the reliability of the data. The intra-observer ICC ranged from 0.95% and 0.96%, and the inter-observer ICC, between 0.97% and 0.99%.
Results
The total range of rotation showed significant differences depending on the protocol (F5, 11 = 61.42; p < .01), of the differences between shoulder and hip (F5, 11 = 335.19; p < .01) and an interaction between the protocol and the shoulder vs. hip factor (F5, 11 = 18.45; p < .01). Shoulder rotation was greater than hip rotation in the six protocols studied (Figure 2A) (F5, 11 = 61.422; p < .01). The lowest values were obtained for both cases in AEBR (p < .00; p = .01), while they were similar in BR, ACBR and ACBL (Table 1). On the NEA side, shoulder rotation was greater than hip rotation for the six protocols studied (F5, 11 = 395.402; p < .01). The highest value of rotation in shoulders and hips was obtained in AEBL (Table 1). On the EA side, the rotation of the shoulders was greater than that of the hips in BH, BR, ACBR and ACBL (F5, 11 = 154.336, p < .01), but was similar to hips in AEBR and AEBL (Table 1).


* Statistical differences between the shoulder and hip (p < .05). ? Statistical differences to BH (p < .05).
Significant differences in shoulder rotation symmetry were shown in all protocols (F5, 11 = 60.151, p < .01) between EA side and NEA side (F5, 11= 379.238; p < .01), except in BH (Table 1). Similar differences were found in the symmetry of hip rotation (F5, 11 = 18.745; p < .01) comparing the EA side with the NEA side (F5, 11 = 279.26; p < .01). In particular, the asymmetry in the BR, AEBR and AEBL protocols achieved more rotation on the NEA side, in both shoulders and hips, while the asymmetry in ACBR and ACBL achieved more rotation on the EA side (Table 1). Total stroke time was greater in ACBR (p = .02) and ACBL (p = .01) compared to the other protocols (F5, 11 = 5.75, p < .01) (Figure 2B). Rotation at the shoulders and hips followed a similar trajectory, although the hips took longer than the shoulders to reach the maximum amount of rotation in all six protocols (F5, 11 = 141.46; p < .01). On the EA side, in the BH, BR and ACBR protocols, the maximum rotation of the shoulders and hips was obtained later in the total stroke duration compared to the AEBR and AEBL (F5, 11 = 6.83; p = .04).
Discussion
The objective of this study was to determine the differences in shoulder and hip rotation caused by different front crawl exercises and the symmetry of that rotation. Our results showed that breath-holding (which acted as a control) achieved shoulder and hip rotation symmetrically on both sides, while asymmetry was observed in all protocols when breathing, as rotation values increased in ~7-12º on the non-breathing side (NEA). Swimming exercises that allowed the arm to be extended forward produced a reduction in the range of total rotation, at both the shoulders and the hips. However, there were greater asymmetries between EA and NEA, with a notable rotation towards the NEA side. In the swimming exercises with the arm close to the body, the ranges of total rotation and asymmetry were similar to those for normal conditions, although a greater rotation was achieved on the EA side, which was the opposite of that found in the other protocols. Therefore, swimming exercises altered shoulder and hip rotation, especially when breathing.
Rotation was greater in all protocols on the side opposite the one on which the breath was taken (NEA). This result was expected, given that asymmetries of body rotation have been considered as a way of adapting to breathing (Psycharakis & Sanders, 2010). In fact, it is important to bear in mind that the duration of the breathing action could also modify the traction cycle of the arm and the magnitude of the torque applied on the EA side, since the arm leaves the water before the breath (Psycharakis & McCabe, 2011). According to the buoyancy model proposed by Yanai (2004), the recovery actions of the arms are enough to drive the rotation of the body on the opposite side. Therefore, a recovery action that includes breathing would increase that buoyant torque towards the centre of mass of the body and, therefore, its rotation. Our results agree with previous studies; Payton et al. (1999) found a rotation of 62 ± 4º and 55 ± 4º between breathing and non-breathing conditions. López-Contreras & Arellano (2002) found values of 60º and 48º, while Psycharakis & McCabe (2011) found values of 59º and 51º for the tests with and without breathing. Therefore, it appears that the difference in rotation in swimming between breathing and breathing holding tends to be ~10 ± 3º greater when breathing.
The swimming exercises with the NEA extended (AEBR and AEBL) were the ones that most altered the rotation. The total rotation range was lower than the means obtained in other protocols (Figure 2A), but a significantly lower rotation was achieved on the EA side (~8-19º) compared to the values obtained in the other protocols (~50-60º). This was explained mainly by the fact that the extended arm restricted the rotation of the body, which caused the swimmer’s body to lie flat in the water (Figure 1). In contrast, rotation was significantly greater on the NEA side, producing a large asymmetry relative to the EA side. This difference in asymmetry was particularly large for the AEBL (50-59º difference). Possibly due to differences in laterality dominance or neck flexibility, swimmers exaggerated a movement they were not used to (Psycharakis & Sanders, 2008). In any event, some authors have stated that this position can place the EA trajectory in a different position to its usual one, causing the hand to pass well beyond the midline of the body towards the opposite hip (Payton et al., 1999; López-Contreras & Arellano, 2002; Liu et al., 1993). Thus, if swimmers do not compensate for changes in body rotation, the stroke may not be able to reach its optimal depth and its propulsion effectiveness could be compromised (Lecrivan et al., 2010; Liu et al., 1993).
On the other hand, these changes could be greater if the frequency of the strokes is not kept constant, since an excessive increase in the actions of the EA could cause a shortening of the stroke, thus reducing its propulsive capacity (Cuenca-Fernández, 2020a; Funai et al., 2019). When considering the stroke cycle time (Table 1), the AEBR and AEBL protocols did not give any significant differences to the normal swimming time, so it cannot be confirmed that the propulsive efficiency could have been reduced with a faster stroke. However, this possibility should not be completely ruled out by coaches when including this exercise. Additionally, it is important to note that repetitive overhead positioning of the arms, coupled with forced adduction and internal rotation of the shoulders, could lead to shoulder impingement and rotator cuff tendinosis, and ultimately could cause shoulder pain or instability and incorrect swimming technique. Therefore, this exercise should be considered with caution if shoulder impingement of the outstretched arm is to be avoided (Yanai & Hay, 2000).
The ACBR and ACBL protocols offered rotation values similar to those found in BH and BR (Figure 2A). However, because one arm was immobile next to the body and breathing was on that side, the body needed to create additional rotation to bring the head into a position out of the water that would allow breathing. This caused a unique increase in rotation on the EA side, at both the shoulders and hips, and explained why the angle of rotation was greater on the EA side than on the NEA side. López-Contreras & Arellano (2002) studied underwater front crawl trajectories that restrict one arm and showed that the time spent in the first part of the stroke (i.e. extension and catch) was reduced in favour of adopting a body position that allows breathing. Consequently, swimmers increased the duration of the hand-thrusting motion to create tangible propulsion, but also as a way to produce enough body rotation to the opposite side to release that arm from the water (Figure 1). However, the fact that the ACBR and ACBL protocols had the longest stroke cycle times (Table 1), possibly indicated that either i) no time was wasted on extension and catch, or ii) a higher than normal resistance was produced by the positioning of the body. The propulsion of the swimmer depends on the hydrodynamic forces created by the movements of their extremities, therefore the application of propulsive forces with a single arm could increase the inertial forces to overcome the resistance of the water, decreasing the propulsive capacity and the swimming speed (Cuenca-Fernández et al., 2020a; Cuenca-Fernández et al., 2020b; Marinho et al., 2009). Further, this speed would be offset by the resistance imposed by the front surface, as the position with one arm extended produces 12.5% more resistance than the sliding position with both arms extended, and this quotient rises to 21.5% in the position with both arms close to the body (Vorontsov & Rumyantsev, 2000; Marinho et al., 2009). Therefore, apart from lower efficiency when using only one arm, this exercise would offer more resistance in the water, which would produce a lower swimming speed.
Temporal analysis showed that the shoulders reached maximum rotation earlier than the hips, with total cycle time values of ~86-89% for shoulders and ~89-92% for hips on the NEA side; and values of ~35-40% and ~37-44% for the EA side, respectively (Figure 2B). This agrees with the study by Psycharakis & Sanders (2008), who showed values of 78.3 ± 7.3% of total cycle time for shoulders and 79.7 ± 9.6% for hips on the side opposite the breathing side (i.e., the NEA side). Considering the total rotation achieved by the shoulders and hips (Figure 2A), the rotation of the hips on the NEA side (~40-60º) was less than that of the shoulders (~50-80º), but the rotation of the hips was performed over a longer time than that of the shoulders. In any case, while the AEBL produced the greatest shoulder rotation, this increase was also accompanied by a marked increase in rotation at the hips (Table 1). This supports the theory that longitudinal rotation can be transferred from the shoulder to the hips (Andersen et al., 2020; Sanders & Psycharakis, 2009). For the ACBR and ACBL protocols, the increase in shoulder rotation on the side opposite the breathing side (EA side) was consistent with the increase found at the hips on the same side of the body. Therefore, according to other authors (Yanai, 2001; Psycharakis & Sanders, 2008), increased shoulder rotation increased hip rotation, but the hips rotated less, and later, than the shoulders. Thus, we deduce that shoulders and hips followed a parallel trajectory, but they did not achieve the same range of motion nor did they do so at the same time (Andersen et al., 2020; Cappaert et al., 1995; Yanai, 2003).
This study shows the limitation of not having measured the trajectory of the hand using 3D techniques, which could have provided an accurate approximation of how changes in body rotation could generate different hand trajectories. In addition, future studies should observe how the rotation of the anteroposterior axis is modified with swimming exercises from a zenithal point of view, since this rotation could increase water resistance. In any case, since shoulder and hip rotation affect joint movements and paraspinal muscle development, the impact of these exercises on the swimmer’s body could improve health depending on personal goals and should also be considered for back ailments.
Conclusions
Swimming exercises with one arm extended produced a high asymmetry in body rotation during breathing and modified the body position during the stroke. Swimming exercises with the arm close to the body were the most similar in terms of the general rotation of the body while swimming front crawl. However, these exercises generated changes in the stroke cycle time that indicated that the swimmer’s technique worsened due to the body positioning. Therefore, its transference could be questionable. In any case, it is important to keep in mind that the effects that these exercises can produce depend on the time, intensity and frequency with which the instructors apply them, so their long-term effects have yet to be determined.
Acknowledgements
This study was supported by a grant from the Ministerio de Ciencia, Innovación y Universidades (Agencia Española de Investigación) and the European Regional Development Fund (ERDF); PGC2018-102116-B-I00 “SWIM II: Innovative measures specific to water: Applied to performance improvement”.
References
[1] Andersen JT., J.T., Sinclair, P.J., McCabe, C.B., & Sanders, R.H. (2020). Kinematic Differences in Shoulder Roll and Hip Roll at Different Front Crawl Speeds in National Level Swimmers. J Strength Cond Res. 2020;, 34(1):), 20-5. DOI:25. https://doi.org/10.1519/JSC.0000000000003281
[2] Bielec, G., Makar, P., & Foliñski, P. (2008). Biomechanical effects of application of the technique exercises in young swimmer training. Wroclaw: Science in Swimming II. 2008:, 51-959.
[3] Cappaert, J.M., Pease DL., & Troup J.P. (1995). Three-Dimensional Analysis of the Men‘s 100-m Freestyle during the 1992 Olympic Games. J Appl Biomech. 1995;, 11(1):), 103-12. DOI:112. https://doi.org/10.1123/jab.11.1.103
[4] Cuenca-Fernández, F., Ruiz-Navarro, J.J., & Arellano Colomina, R, editors. (2020a). Strength-velocity relationship of resisted swimming: A regression analysis. ISBS-Conference Proceedings Archive. 2020a, 38(1), 99.
[5] Cuenca-Fernández, F., Gay, A., Ruiz-Navarro JJ., Morales-Ortíz, E., López-Contreras, G., & Arellano, R. (2020b). Swimming performance after an eccentric post-activation training protocol. Apunts Educación Física y Deportes, 140, 44-51. https://doi.org/10.5672/apunts.2014-0983.es.(2020/2).140.07
[6] de Souza-Castro, F.A., Villas-Boas JP., & Stringhini-Guimarães., A.C. (2007). Effects of swimming intensity and breathing in front crawl body roll angles for swimmers and triathletes. Brazilian J Biomech. 2007; 7(13):), 85-90.
[7] Funai, Y., Matsunami, M., & Taba, S. (2019). Physiological Responses and Swimming Technique During Upper Limb Critical Stroke Rate Training in Competitive Swimmers. J Hum Kinet. 2019,70(1), 61-8. DOI:68. https://doi.org/10.2478/hukin-2019-0026
[8] Lecrivain, G., Payton, C., Slaouti, A., & Kennedy, I. (2010). Effect of body roll amplitude and arm rotation speed on propulsion of arm amputee swimmers. J Biomech. 2010;, 43(6), 1111-7. DOI:1117. https://doi.org/10.1016/j.jbiomech.2009.12.014
[9] Liu, Q., Hay J.G., & Andrews, J.G. (1993). Body roll and handpath in freestyle swimming: an experimental study. J Appl Biomech. 1993, 9(3), 238-53. DOI:253. https://doi.org/10.1123/jab.9.3.238
[10] López-Contreras, G., & Arellano, R. (2002). Análisis del efecto de las modificaciones de la ejecución del estilo crol en la rotación longitudinal del cuerpo. Colección icdICD: investigación en ciencias del deporte. 2002, (32):), 183-218.
[11] Lucero, B. (2015). The 100 best swimming drills: (3rd ed.). Meyer & Meyer Verlag; 2015.
[12] Marinho D.A., Reis V.M., Alves F.B., Vilas-Boas J.P., Machado, L., Silva A.J., & Rouboa, A.I. (2009). Hydrodynamic drag during gliding in swimming. J Appl Biomech. 2009;, 25(3), 253-7. DOI:257. https://doi.org/10.1123/jab.25.3.253
[13] Payton, C.J., Bartlett, R.M., Baltzopoulos, V., & Coombs, R. (1999). Upper extremity kinematics and body roll during preferred-side breathing and breath-holding front crawl swimming. J SportSports Sci. 1999;, 17(9), 689-96. DOI:696. https://doi.org/10.1080/026404199365551
[14] Payton, C.J., Hay J.G., & Mullineaux D.R. (1997). The effect of body roll on hand speed and hand path in front crawl swimming: a simulation study. J Appl Biomech. 1997, 13(3), 300-15. DOI:315. https://doi.org/10.1123/jab.13.3.300
[15] Prichard, B. (1993) A new swim paradigm: Swimmers generate propulsion from the hips. Swimming Technique. 1993, 30(1),17-23.
[16] Psycharakis S.G., & McCabe, C. (2011). Shoulder and hip roll differences between breathing and non-breathing conditions in front crawl swimming. J Biomech. 2011, 44(9), 1752-6. DOI:1756. https://doi.org/10.1016/j.jbiomech.2011.04.004
[17] Psycharakis S.G., & Sanders R.H. (2008). Shoulder and hip roll changes during 200-m front crawl swimming. Med Sci Sport Exer. 2008; Sports Exerc, 40(12), 2129-36. DOI:2136. https://doi.org/10.1249/mss.0b013e31818160bc
[18] Psycharakis, S.G., & Sanders, R.H. (2010). Body roll in swimming: A review. J SportSports Sci. 2010, 28(3), 229-36. DOI:236. https://doi.org/10.1080/02640410903508847
[19] Sanders, R.H., & Psycharakis, S.G. (2009).Rolling rhythms in front crawl swimming with six-beat kick. J Biomech. 2009, 42(3), 273-9. DOI:279. https://doi.org/10.1016/j.jbiomech.2008.10.037
[20] Vorontsov, A., & Rumyantsev, V. (2000). Propulsive forces in swimming. In V. Zatsiorsky (Ed.), Biomechanics in sport: Performance, enhancement and injury prevention. 2000:, (pp. 205-31.231). Oxford: Blackwell Science Ltd. https://doi.org/10.1002/9780470693797.ch10
[21] Wen, Y., Peng, Y., Zhao, F., & Zhen, K. (2016). The effects of specific drills on the flip turns of freestyle swimmers based on a kinesiology analysis. J Hum Kinet. 2016, 52(1), 165-73. DOI:173. https://doi.org/10.1515/hukin-2016-0004
[22] Yanai, T. (2001) What causes the body to roll in front-crawl swimming? J Appl Biomech. 2001, 17(1), 28-42. DOI: https://doi.org/10.1123/jab.17.1.28
[23] Yanai, T. (2003). Stroke frequency in front crawl: its mechanical link to the fluid forces required in non-propulsive directions. J Biomech. 2003, 36(1), 53-62. DOI: https://doi.org/10.1016/S0021-9290(02)00299-3
[24] Yanai, T. (2004). Buoyancy is the primary source of generating bodyroll in front-crawl swimming. J Biomech. 2004, 37(5), 605-12. DOI:612. https://doi.org/10.1016/j.jbiomech.2003.10.004
[25] Yanai, T., & Hay JG., J.G. (2000). Shoulder impingement in front-crawl swimming: II. Analysis of stroking technique. Med Sci Sport Exer. 2000; Sports Exerc, 32(1), 30. DOI: https://doi.org/10.1097/00005768-200001000-00006
[26] Zhang, P..(2014). Basic Land Drills for Swimming Stroke Acquisition. JTRM in Kinesiology. 2014, 1-16
ISSN: 2014-0983
Received: July 2, 2021
Accepted: October 28, 2021
Published: April 1, 2022
Editor: © Generalitat de Catalunya Departament de la Presidència Institut Nacional d’Educació Física de Catalunya (INEFC)
© Copyright Generalitat de Catalunya (INEFC). This article is available from url https://www.revista-apunts.com/. This work is licensed under a Creative Commons Attribution-NonCommercial-NoDerivatives 4.0 International License. The images or other third party material in this article are included in the article’s Creative Commons license, unless indicated otherwise in the credit line; if the material is not included under the Creative Commons license, users will need to obtain permission from the license holder to reproduce the material. To view a copy of this license, visit https://creativecommons.org/licenses/by-nc-nd/4.0/deed.en