Cardiocirculatory, metabolic, and perceptual responses in elite wheelchair fencing competition
*Corresponding author: Laura Ruiz-Sanchis laura.ruiz@ucv.es
Cite this article
Martín-Ruiz, J., Alarcón-Jiménez, J., de-Bernardo, N., Iglesias, X. & Ruiz-Sanchis, L. (2024). Cardiocirculatory, metabolic and perceptive responses in elite wheelchair fencing competition. Apunts Educación Física y Deportes, 157, 51-57. https://doi.org/10.5672/apunts.2014-0983.es.(2024/3).157.06
Abstract
Wheelchair fencing is a sport characterized by intermittent bursts of effort in which the speed of actions is at its maximum. However, there is a lack of literature concerning the evolution of fatigue in this sport when compared to the available information related to the Olympic discipline. The objective of this study was to calculate the cardiocirculatory, metabolic, and perceptual values in an international wheelchair fencing competition in order to enhance the resources for specific training for this sport. A total of 16 fencers from categories A (7) and B (9) competed. The participants were monitored for heart rate, blood lactate, and perceived exertion during each phase of the competition. Both heart rate (p = .014) as well as blood lactate (p = .037) and perceived exertion (p = .04) increased more in the direct elimination phase than in the pool phase and stabilized during the final portion of the competition. In conclusion, all the parameters analyzed tended to increase after the pool phase and reached their maximum values during direct elimination bouts. Despite the metabolic values slightly exceeding the aerobic threshold, there is a need to create training strategies to delay fatigue, and thereby prevent coordination errors, as preventing said errors is particularly important in this Paralympic discipline, as it requires heightened accuracy at maximum speeds.
Introduction
Olympic fencing is an acyclic sport with an elevated number of high-speed actions. In terms of energy demand, in a competition involving multiple bouts within a total interval of 3-4 hours, the aerobic system contribution is about 80-90% (Bottoms et al., 2023). This aspect is more pronounced in direct eliminations, with an estimated energy expenditure of 8.6 ± 0.54 metabolic equivalents (Milia et al., 2014); therefore, improving this system, exemplified by adaptations such as increased size and number of mitochondria, will help maintain intensity over a longer period (Yang et al., 2022).
This sport is of an intermittent nature, demanding a vast amount of energy from the phosphagen system (Turner et al., 2014). From an analytical perspective, alactic anaerobic stimuli occur during a bout due to a series of actions within a unit of time, with blood lactate values reaching 4 mmol·L-1 (Turner et al., 2017b), bearing in mind the initial pool classification rounds and the following direct elimination rounds, until the end of the competition, in which the difficulty of the rivals increases.
Calculating the intensity at which a fencer competes from a cardiorespiratory perspective is key to planning their physical training. While the demands of a wheelchair competition are lower than those of standing fencing, and similar in direct elimination bouts (Iglesias et al., 2019), it is not comparable to other wheelchair sports like basketball (Vaquera et al., 2016) due to the type of exertion that each entail.
Taking Olympic fencing as a reference, energy input in absolute terms (Passali et al., 2015) indicates that the contribution of the aerobic system is very similar across the various competition phases (pools: 185.55 ± 36.43 kJ; 1st elimination bout: 185.46 ± 28.81 kJ 2nd elimination bout: 186.62 ± 27.70 kJ). In the case of phosphagens, a higher contribution occurs in pools (28.70 ± 10.09 kJ) than in direct elimination rounds (1st: 19.53 ± 9.49 kJ; 2nd: 22.25 ± 9.27 kJ). Lastly, the glycolytic system is far superior in pool rounds (16.42 ± 6.47 kJ) as compared to the following 2 rounds (1.20 ± 1.32 kJ and 1.34 ± 1.84 kJ, respectively).
In terms of relative energy, the aerobic system contributes less (p < .05) in pool bouts (80.57 ± 4.45%) than in comparison to the first and second 15-touch direct elimination bouts (90.02 ± 4.69% and 88.8 ± 4.28%), respectively. On the other hand, the glycolytic system takes on greater prominence in the first round (6.97 ± 2.53%) than in the following two rounds (0.63 ± 0.73% and 0.60 ± 0.80%). In the case of phosphagens, these remain stable with some fluctuation (pools: 12.44 ± 6.67%; 1st direct bout: 9.35 ± 4.30%; 2nd direct bout: 10.60 ± 4.52%) (Franchini, 2023).
The unit of measure typically used for taking measurements during these competitions is related to the heart rate reserve and the level of VO2 max., an aspect challenged by various authors who indicate that the latter value is overestimated (for example in sports such as cycling or running) and should therefore be carefully considered (Guimarães et al., 2019). This has been confirmed by other researchers who indicate that this correlation is individual and due to intrinsic characteristics that cannot be generalized (Ferri Marini et al., 2022), as benefits from other capacities may influence this, such as the fact that strength training may contribute to improving strength resistance in combat sports (Cid-Calfucura et al., 2023).
In this respect, there is indeed a consensus regarding the use of perceptual and heart rate values, given the proven high correlation between perceived exertion and beats per minute after exercise (r = .84- .98), during exercise (r = .73 – .85), and during its respective phases (r = .82-.92) (Turner et al., 2017a).
Scales have been shown to be good indicators of intensity, even in strength training exercises, for which the correlation sits at r = .8 (Lagally & Costigan, 2004), even for the precision assessment of the impact of an activity in individuals in wheelchairs (Zhao et al., 2022), and therefore have been shown to be a reliable system for regulating intensity in this capacity. Models such as the OMNI-RES picture system scale can be used in strength training exercises by both men and women. When strength increases, this is reflected in the scale items, which vary according to the exercise (Gearhart et al., 2009). This is relevant because it gives trainers or coaches tools to indicate areas for improvement according to the physiological systems involved (Lagally et al., 2009).
With this in mind, it should be possible to create a competition strategy that addresses fatigue and allows for the incorporation of resources that enable the onset of said fatigue to be delayed, as occurs with nutritional resources: mouth rinsing carbohydrate solution enables skill level to remain the same, though it does not have the same effect on reaction speed in high-level fencers (Rowlatt et al., 2017). In this regard, dehydration is common and represents a very significant risk, though more so in men than in women. This means fluid replacement is fundamental, otherwise pulse is impacted and subsequently rises (Eda et al., 2022), even if it does not have any direct effects on lactate levels (Chryssanthopoulos et al., 2020).
In addition, Olympic and Paralympic fencing have three different disciplines (épée, foil, and saber), with differing action and resting times that also require different energy substrate demands (Tarragó et al., 2023). Some studies have focused on analyzing simulated competitions; for example, in simulated foil competitions, higher beats per minute and ratings of perceived exertion (RPE) values have been observed in direct elimination bouts (163 ± 13 bpm-1 and 3.7 ± 1.2 RPE in pool and 170 ± 10 bpm-1 and 5.6 ± 1.6 RPE in direct elimination) (Bottoms et al., 2023). Similar values have been observed in simulated épée competition, but with fewer differences between elimination rounds (168 ± 12 bpm-1 in and 169 ± 14 bpm-1 in direct elimination) (Oates et al., 2019). Assessments during official competition have not been widely studied in the literature, however heart rate and blood lactate levels have been described in men’s épée bouts with values of 166 ± 8 bpm-1 and 3.2 ± 0.7 mmol·L-1 , and in women’s foil bouts, with values of 173 ± 7 bpm-1 and 4.2 ± 0.9 mmol·L-1 (Iglesias & Rodríguez, 1995).
The current literature has little specific data on wheelchair fencing in competition settings. One of the most recent studies described oxygen consumption values in training bouts of 23.3 ± 6.1 ml·min-1·kg-1 with peak values of 32.1 ± 7.9 ml·min-1·kg-1. The heart rates recorded during these training bouts corresponded to a mean intensity of 72.7 ± 10.3% of the fencers’ maximum heart rate. Likewise, the recorded lactate levels were 3.5 ± 3.6 mmol·min-1 and RPE was 4.8 ± 3.2 (Oates et al., 2019).
Given the scarce diversity of studies with which to establish a comparison for the quantification of energy output and perception in official competition wheelchair fencing, the aim of this study was to focus on calculating cardiocirculatory (heart rate, heart rate reserve [Bok et al., 2023], WINT index [Sowan et al., 2023]), metabolic (blood lactate), and perceptual values over the course of an international competition with participation from elite category A and B athletes from Finland, Hungary, Peru, Romania, and Spain. Categories A and B correspond to the functional classifications for Paralympic fencing competition.
These calculations will help identify the training loads for these types of fencers and optimize competition-focused physical training programs to counteract fatigue while also contributing to more in-depth knowledge about this Paralympic sport.
Methodology
Participants
An international wheelchair fencing tournament was organized with the épée chosen as the weapon. Within the tournament, 3 different competitions were held with the total number of participants (n = 16): i) one with category A athletes (n = 7), these being the most functional with good sitting balance, trunk mobility, and good control of their fencing arm; ii) a second with category B athletes (n = 5), with impairment of the legs, trunk, or fencing arm, and iii) a third and last competition with less-experienced category B athletes (n = 4). The participating countries were Hungary (1), Finland (2), Romania (1), Peru (1), and Spain (11). The sample characteristics are described in Table 1.

The study was approved by the Research Ethics Committee (REC) of the Catholic University of Valencia with Resolution No. UCV/2022-2023/107 and was based on the guidelines described in the Declaration of Helsinki regarding the ethical principles for medical research involving human subjects, and all the individuals participating in the study signed the corresponding informed consent.
Materials and methods
Each athlete underwent two specific anthropometric measurements: calculation of seated height and arm span. Neither of the regular height and weight measurements were taken due to the impossibility of taking them in most cases.
For seated height, the participants sat on a 44 cm-high stool. Sitting upright, the head was maneuvered into the Frankfort angle and the stadiometer (Seca 213, Hamburg, Germany) was placed on the vertex of the head. The height of the stool was subtracted from the result to obtain the final height.
To measure arm span, the subject was seated on a backless stool against a wall with the hips and shoulders touching the wall. The subject was instructed to perform a shoulder abduction and elbow extension with the fingers extended. The maximum length between the middle fingers of both hands was measured.
Heart rate (HR), heart rate reserve (HRR), WINT aerobic index (WI), and RPE data were analyzed to determine the fatigue accumulated over the course of a competition. For the physiological values, heart rate was monitored during the competition using a coded armband heart rate sensor (Moofit, Shenzhen, Guangdong, China), and the data were collected using the Pulsemonitor software (Pulsemonitor, Michalowice, Polaned). With the previously recorded age and baseline heart rate data, heart rate reserve was obtained using the following equation:
And the WINT aerobic load index using the calculation below:
An épée competition was held according to the regular phases: an initial round of 5-touch, 3-minute pool bouts and a second phase of 15-touch, 9-minute direct elimination bouts. To measure metabolic stress, after each phase the participants’ lactate levels were measured 3 minutes after the end of each bout, performed on the earlobe using the Lactate Pro2 analyzer (Arkray Inc., Kyoto, Japan).
While these samples were being taken, RPE was recorded at each interval using the OMNI-RES scale from Robertson et al. (2003), with the aim of establishing a correlation between physiological and perceptual indicators. These procedures are summarized in Figure 1.
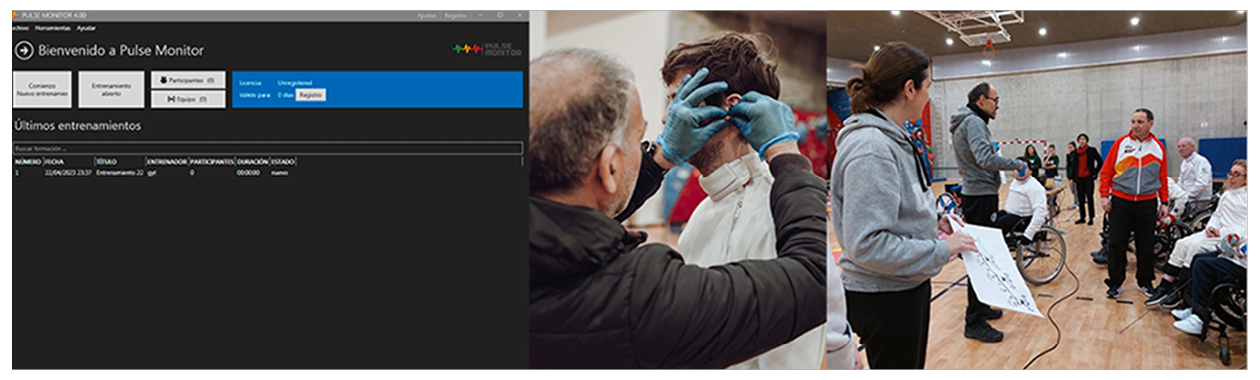
Statistical analysis
The data were described using means and standard deviation, as well as medians and interquartile ranges for the continuous quantitative variables and using proportions for the qualitative variables.
The model was adjusted as per the Zuur and Ieno protocol (2016). First, the data structure error was determined by adjusting beyond the optimal model. In the case of the models for determining changes in heart rate and lactate accumulation, the model was adjusted as follows:
Y~Phase*(Experience+Category+Sex)+1(1|ID)
For the model used to determine changes in perceived exertion, this was adjusted in the following manner:
Y~Phase*(Experience+Category+Sex)+HR+Lactate+(1|ID)
This allowed for consideration of all the factors studied and the first-level interactions between the sociodemographic factors and the competition phase.
Second, the random effects model was adjusted using the individual (ID), estimating the coefficients with the restricted maximum likelihood (REML) method. Subsequently, the fixed effects model was adjusted with the ML method, repeatedly eliminating interactions until the model could no longer be improved. Lastly, the optimal model was generated estimating the coefficients with the REML method. To interpret the model, the coefficients and the results of the type II ANOVA were considered. For the significant categorical factors, post-hoc comparisons were made using the emmeans package.
All the nested models were compared among themselves using the corrected Akaike information criterion (AICc). In all cases, the assumptions of the linear models were checked, visually inspecting the residuals and the DHARMa residuals. The models were adjusted using the lme4 v.1.1-30 and lmerTest v.3.1-3 packages.
All the analyses were carried out with R (R Core Team, 2013) v.4.2.2. The openxlsx v4.2.5 package was used to read the data tables (for xlsx files) and/or the haven v.2.5.0 package (for sav files). The graphs were created using ggplot2 v.3.3.6 ggpubr v.0.4.0 and other functions included in the previously mentioned programs. For all the analyses, α = .05 was used.
Results
The initial heart rate values prior to competition were 87.88 ± 23.29 bpm-1 and lactate values 2.36 ± 0.84 mmol·L-1. Figure 2 shows the evolution of heart rates across each of the phases and how heart rate values were significantly higher in the direct elimination phases, but without any differences between the sexes (p = 605). Tukey’s test indicated significant changes between both phases of the competition (p = .014). These data present a high correlation with two variables dependent on this value, in the case of heart rate reserve r = .90 and the WINT aerobic load index, r = .76.
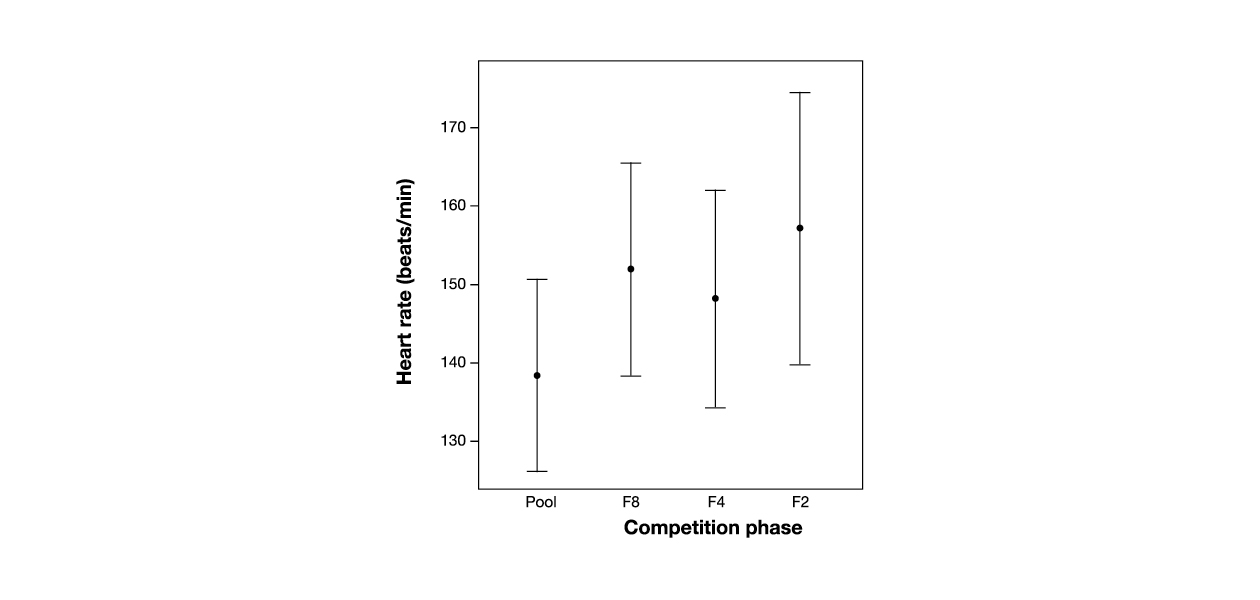
Table 2 shows the difference in lactate accumulation between the pool phase and direct elimination phases. As with the heart rate, there were no differences between men and women (p = .792).

Figure 3 shows the differences in perceived exertion in pool bouts versus the rest of the tournament (F8, F4, F2). A significant effect was detected indicating that perceived exertion was 1.34 ± 0.43 points higher in the competition phases than in the previous phase.
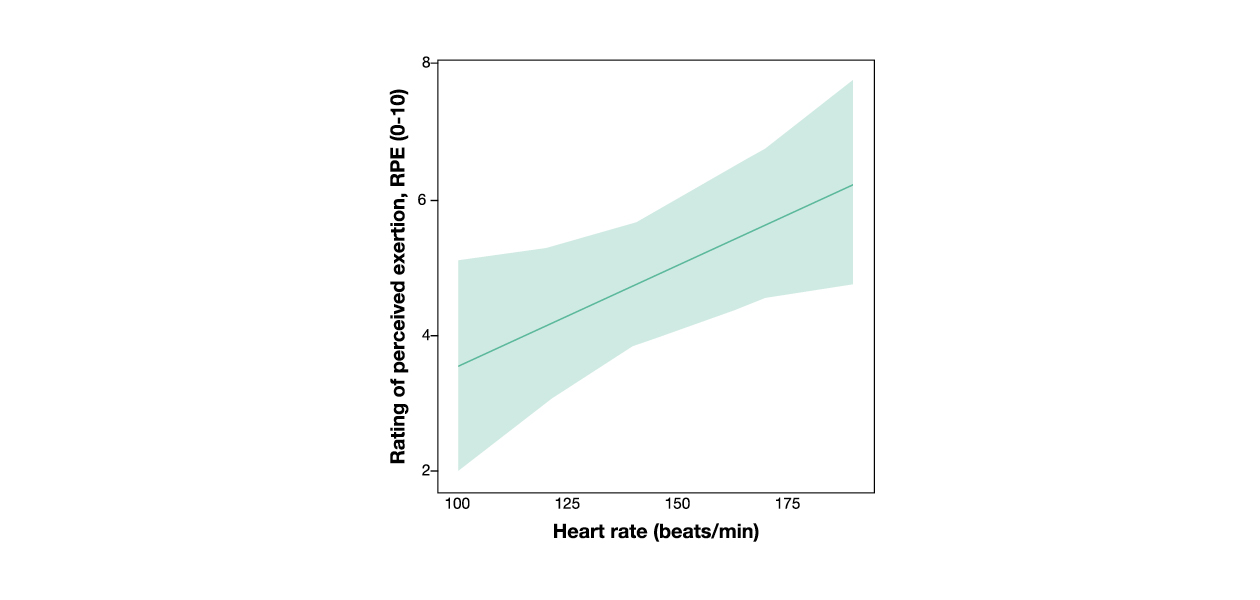
Bearing in mind the different competition phases, significant differences were observed between the initial phase and subsequent phases (p = .028 and p = .002), though this significant increase did not occur between phases F4 and F2 nor were any differences between the sexes reported (p = .535).
Discussion
It is important to identify the physiological responses resulting from real competition settings, as this helps define the training needs of athletes.
The characteristics of each of the bouts were similar to an interval training exercise, wherein there is a high degree of uncertainty due to the intermittent pattern. In settings in which measuring respiratory parameters such as VO2 max. is not possible, having cardiocirculatory, metabolic, and perceptual records is sufficient (López-Chicharro & Vicente-Campos, 2018) for detecting and subsequently addressing the resulting needs for improvement. Along this line of reasoning, Iglesias et al. (2023) presented a study on wheelchair fencing in which they state that it is possible to use heart rate to estimate oxygen consumption by assuming a certain degree of underestimation (9.9%) but with good correlation indices (r = .843, p < .001).
Over the course of the competition, the average heart rate was 14.08 ± 5.33 beats higher in the direct elimination than in the pool phases, thereby confirming that reported by Turner et al. (2017b), who indicated that this phase, with 15 touches per bout, conditions the contrast for this value (p = .014) between phases, only to later stabilize until the end of the competition (F8-F4 p = .875, F8-F2 p = .830, F4-F2 p = .561). This finding shows that both Olympic and Paralympic fencing present similar behaviors in terms of cardiocirculatory response. Fatigue increases progressively in both disciplines and presents a high correlation with heart rate reserve (r = .90) and the WINT aerobic load index (r = .76). This analysis should be taken into consideration in relation to respiratory rate, which increases with respect to other physical exercises, given that nasal airway resistance following a bout is 0.28 ± .16 Pacm3/s. Without it, in normal physical exercise, the mean value was 0.24 ± .15 Pacm3/s,meaning significant differences were observed between using or not using a fencing facemask before and after sporting activities (p < .05) (Passali et al., 2015).
In terms of the metabolic profile, there was no significant difference in lactate accumulation between phases (p = .656): a marginally significant effect was observed between the first and second phase of the competition (F8-F4) (p = .037). When analyzing the effect of the competition phase on lactate accumulation, this was greater in the last portion of the competition than the first. This data is in line with that described in Olympic fencing competition (Turner et al., 2017b), in which values exceeding 4 mmol·L-1 were recorded in the final portion of the tournament.
Likewise, no significant variations (p = .089) were observed when considering the category: category A accumulated slightly more than B (1.307 ± 0.715). It is important to bear in mind that more experience correlated with less increase (reduction of 0.127 ± 0.064 mmol·L-1), which could be related to the fact that elite fencers are capable of generating greater speeds than lower-level fencers, despite increases in their blood lactate (Weichenberger et al., 2012) compared to those lower-lever athletes. This is important because the resulting peripheral fatigue comes with coordination impairment that can inhibit precision when performing technical movements requiring maximum speed (Varesco et al., 2023).
The RPE followed a similar path as heart rate. This increased as the competition progressed and was most notable between pools and direct elimination bouts (p = .004) and each of the subsequent phases (F8-F4 p = .028; F8-F2 p = .002), with the exception of the last one (F4-F2 p = .212). This increase occurred in a parallel manner to that of heart rate (.03 ± .014 for each heart rate unit). This confirms the relationship between perception and heart rate, which in Olympic fencing has obtained a very high correlation during competition (r = .82-92) (Turner et al., 2017a), confirming that direct elimination bouts require greater physiologicaldemand (Bottoms et al., 2023). In this regard, no consistent relationship between subjective perceived exertion and increase in blood lactate has been found.
Conclusions
The main conclusions that can be gathered from the cardiocirculatory responses are that the highest heart rate recordings occur in direct elimination phases and these stabilize towards the end of competition. In terms of the metabolic profile, no differences in lactate accumulation occur between phases, rather a gradual increase can be observed. Lastly, perceptual values increase progressively over the course of a tournament. Understanding the responses that occur during real wheelchair fencing competitions make it possible to adapt training programs to athletes’ specific physical and technical/tactical needs without the need to make adaptations to the Olympic fencing discipline, as has been done until now.
Funding
This study received funding of €6,500 from the project “Physiological Variables of Wheelchair Fencing” (#2023-233-003) from the Catholic University of Valencia and was carried out at the Faculty of Physical Activity and Sport Sciences and Physiotherapy of the Catholic University of Valencia, Spain.
References
[1] Bok, D., Gulin, J., Škegro, D., Šalaj, S., & Foster, C. (2023). Comparison of anaerobic speed reserve and maximal aerobic speed methods to prescribe short format high‐intensity interval training. Scandinavian Journal of Medicine & Science in Sports, 33(9), 1638–1647. doi.org/10.1111/sms.14411
[2] Bottoms, L., Tarragó, R., Muñiz, D., Chaverri, D., Irurtia, A., Castizo-Olier, J., Carrasco, M., Rodríguez, F. A., & Iglesias, X. (2023). Physiological demands and motion analysis of elite foil fencing. PloS One, 18(2), e0281600. doi.org/10.1371/journal.pone.0281600
[3] Chryssanthopoulos, C., Tsolakis, C., Bottoms, L., Toubekis, A., Zacharogiannis, E., Pafili, Z., & Maridaki, M. (2020). Effect of a carbohydrate-electrolyte solution on fluid balance and performance at a thermoneutral environment in international-level fencers. Journal of Strength and Conditioning Research, 34(1), 152-161. doi.org/10.1519/JSC.0000000000003065
[4] Cid-Calfucura, I., Herrera-Valenzuela, T., Franchini, E., Falco, C., Alvial-Moscoso, J., Pardo-Tamayo, C., Zapata-Huenullán, C., Ojeda-Aravena, A., & Valdés-Badilla, P. (2023). Effects of strength training on physical fitness of olympic combat sports athletes: A systematic review. International Journal of Environmental Research and Public Health, 20(4), 3516. doi.org/10.3390/ijerph20043516
[5] Eda, N., Azuma, Y., Takemura, A., Saito, T., Nakamura, M., Akazawa, N., Yamamoto, M., Naito, T., Kasai, N., Shimizu, K., Aoki, Y., & Hoshikawa, M. (2022). A clinical survey of dehydration during winter training in elite fencing athletes. The Journal of Sports Medicine and Physical Fitness, 62(11), 1534-1540. doi.org/10.23736/S0022-4707.21.13288-8
[6] Ferri Marini, C., Sisti, D., Skinner, J. S., Sarzynski, M. A., Bouchard, C., Amatori, S., Rocchi, M. B. L., Piccoli, G., Stocchi, V., Federici, A., & Lucertini, F. (2022). Effect of individual characteristics and aerobic training on the %HRR-%V̇O2R relationship. European Journal of Sport Science, 23, 1600-1611. doi.org/10.1080/17461391.2022.2113441
[7] Franchini, E. (2023). Energy system contributions during olympic combat sports: A narrative review. Metabolites, 13(2), 297. doi.org/10.3390/metabo13020297
[8] Gearhart, R. F., Lagally, K. M., Riechman, S. E., Andrews, R. D., & Robertson, R. J. (2009). Strength tracking using the OMNI resistance exercise scale in older men and women. Journal of Strength and Conditioning Research, 23(3), 1011-1015. doi.org/10.1519/JSC.0b013e3181a2ec41
[9] Guimarães, G. C., Farinatti, P. T. V., Midgley, A. W., Vasconcellos, F., Vigário, P., & Cunha, F. A. (2019). Relationship between percentages of heart rate reserve and oxygen uptake reserve during cycling and running: A validation study. Journal of Strength and Conditioning Research, 33(7), 1954-1962. doi.org/10.1519/JSC.0000000000002079
[10] Iglesias, X. & Rodríguez, F.A. (1995). Caracterización de la frecuencia cardiaca y la iactatemia en esgrimistas durante la competición. Apunts Sports Medicine, 32(123), 21-32. www.apunts.org/es-caracterizacion-frecuencia-cardiaca-iactatemia-esgrimistas-articulo-X0213371795055195
[11] Iglesias, X., Rodríguez, F. A., Tarragó, R., Bottoms, L., Vallejo, L., Rodríguez-Zamora, L., & Price, M. (2019). Physiological demands of standing and wheelchair fencing in able-bodied fencers. The Journal of Sports Medicine and Physical Fitness, 59(4), 569-574. doi.org/10.23736/S0022-4707.18.08413-X
[12] Iglesias, X., Tarragó, R., Chaverri, D., Montraveta, J., Muniz-Pumares, D., & Bottoms, L. (2023). Oxygen consumption in wheelchair fencing: Direct assessment and validation of an estimation method: 576. Medicine & Science in Sports & Exercise, 55(9S), 188.
[13] Lagally, K. M., Amorose, A. J., & Rock, B. (2009). Selection of resistance exercise intensity using ratings of perceived exertion from the OMNI-RES. Perceptual and Motor Skills, 108(2), 573-586. doi.org/10.2466/PMS.108.2.573-586
[14] Lagally, K. M., & Costigan, E. M. (2004). Anchoring procedures in reliability of ratings of perceived exertion during resistance exercise. Perceptual and Motor Skills, 98(3 Pt 2), 1285-1295. doi.org/10.2466/pms.98.3c.1285-1295
[15] López-Chicharro, J., & Vicente-Campos, D. (2018). HIIT entrenamiento interválico de alta intensidad. Exercise Physiology and Training.
[16] Milia, R., Roberto, S., Pinna, M., Palazzolo, G., Sanna, I., Omeri, M., Piredda, S., Migliaccio, G., Concu, A., & Crisafulli, A. (2014). Physiological responses and energy expenditure during competitive fencing. Applied Physiology, Nutrition, and Metabolism = Physiologie Appliquee, Nutrition Et Metabolisme, 39(3), 324-328. doi.org/10.1139/apnm-2013-0221
[17] Oates, L. W., Campbell, I. G., Iglesias, X., Price, M. J., Muniz-Pumares, D., & Bottoms, L. M. (2019). The physiological demands of elite epée fencers during competition. International Journal of Performance Analysis in Sport, 19 (1), 76-89. doi.org/10.1080/24748668.2018.1563858
[18] Passali, D., Cambi, J., Salerni, L., Stortini, G., Bellussi, L. M., & Passali, F. M. (2015). Effects of a mask on breathing impairment during a fencing assault: A case series study. Asian Journal of Sports Medicine, 6(3), e23643. doi.org/10.5812/asjsm.23643
[19] R Core Team, 2013. R: A language and environment for statistical computing. R Foundation for Statistical Computing, Vienna. www.R-project.org/
[20] Robertson, R. J., Goss, F. L., Rutkowski, J., Lenz, B., Dixon, C., Timmer, J., Frazee, K., Dube, J., & Andreacci, J. (2003). Concurrent validation of the OMNI perceived exertion scale for resistance exercise. Medicine and Science in Sports and Exercise, 35(2), 333-341. doi.org/10.1249/01.MSS.0000048831.15016.2A
[21] Rowlatt, G., Bottoms, L., Edmonds, C. J., & Buscombe, R. (2017). The effect of carbohydrate mouth rinsing on fencing performance and cognitive function following fatigue-inducing fencing. European Journal of Sport Science, 17(4), 433-440. doi.org/10.1080/17461391.2016.1251497
[22] Sowan, B., Hong, T., Al-Qerem, A., Alauthman, M., & Matar, N. (2023). Ensembling validation indices to estimate the optimal number of clusters. Applied Intelligence, 53(9), 9933-9957. doi.org/10.1007/s10489-022-03939-w
[23] Tarragó, R., Bottoms, L., & Iglesias, X. (2023). Temporal demands of elite fencing. PloS One, 18(6): e0285033. doi.org/10.1371/journal.pone.0285033
[24] Turner, A. N., Buttigieg, C., Marshall, G., Noto, A., Phillips, J., & Kilduff, L. (2017a). Ecological validity of the session rating of perceived exertion for quantifying internal training load in fencing. International Journal of Sports Physiology and Performance, 12(1), 124-128. doi.org/10.1123/ijspp.2016-0062
[25] Turner, A. N., Kilduff, L. P., Marshall, G. J. G., Phillips, J., Noto, A., Buttigieg, C., Gondek, M., Hills, F. A., & Dimitriou, L. (2017b). Competition intensity and fatigue in elite fencing. Journal of Strength and Conditioning Research, 31(11), 3128-3136. doi.org/10.1519/JSC.0000000000001758
[26] Turner, A., James, N., Dimitriou, L., Greenhalgh, A., Moody, J., Fulcher, D., Mias, E., & Kilduff, L. (2014). Determinants of Olympic fencing performance and implications for strength and conditioning training. Journal of Strength and Conditioning Research, 28(10), 3001-3011. doi.org/10.1519/JSC.0000000000000478
[27] Vaquera, A., Villa, J. G., Morante, J. C., Thomas, G., Renfree, A. J., & Peters, D. M. (2016). Validity and test-retest reliability of the TIVRE-basket test for the determination of aerobic power in elite male basketball players. Journal of Strength and Conditioning Research, 30(2), 584-587. doi.org/10.1519/JSC.0000000000001078
[28] Varesco, G., Pageaux, B., Cattagni, T., Sarcher, A., Martinent, G., Doron, J., & Jubeau, M. (2023). Fatigue in elite fencing: Effects of a simulated competition. Scandinavian Journal of Medicine & Science in Sports, 33(11), 2250-2260. doi.org/10.1111/sms.14466
[29] Weichenberger, M., Liu, Y., & Steinacker, J. M. (2012). A test for determining endurance capacity in fencers. International Journal of Sports Medicine, 33(1), 48-52. doi.org/10.1055/s-0031-1284349
[30] Yang, W., Park, J., Shin, Y., & Kim, J. (2022). Physiological profiling and energy system contributions during simulated epée matches in elite fencers. International Journal of Sports Physiology and Performance, 17(6), 943-950. doi.org/10.1123/ijspp.2021-0497
[31] Zhao, H., Nishioka, T., & Okada, J. (2022). Validity of using perceived exertion to assess muscle fatigue during resistance exercises. PeerJ, 10, e13019. doi.org/10.7717/peerj.13019
[32] Zuur, A. F. & Ieno, E. N. (2016). A protocol for conducting and presenting results of regression‐type analyses. Methods in Ecology and Evolution, 7(6), 636-645. doi.org/10.1111/2041-210X.12577
ISSN: 2014-0983
Received: January 23, 2024
Accepted: March 15, 2024
Published: July 1, 2024
Editor: © Generalitat de Catalunya Departament de la Presidència Institut Nacional d’Educació Física de Catalunya (INEFC)
© Copyright Generalitat de Catalunya (INEFC). This article is available from url https://www.revista-apunts.com/. This work is licensed under a Creative Commons Attribution-NonCommercial-NoDerivatives 4.0 International License. The images or other third party material in this article are included in the article’s Creative Commons license, unless indicated otherwise in the credit line; if the material is not included under the Creative Commons license, users will need to obtain permission from the license holder to reproduce the material. To view a copy of this license, visit https://creativecommons.org/licenses/by-nc-nd/4.0/deed.en